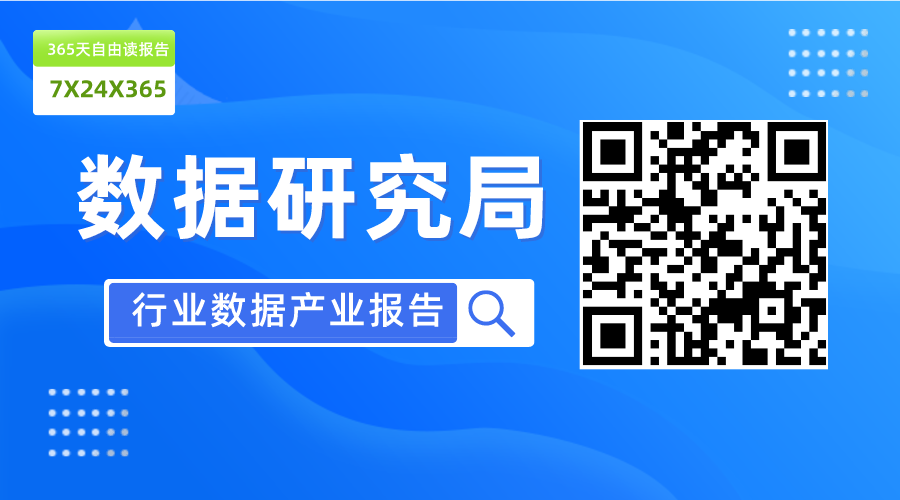
DNV report shipping 2020 shipping 2020 02: shipping 2020 Contents Executive summary 04 Introduction 06 Chapter 1: Trends and drivers 08 The world economy and demand for seaborne transport 10 Environmental regulations 16 Technology and energy efficiency 20 Fuel trends 24 Chapter 2: Future scenarios 26 Why scenarios? 28 Scenario A: “Full steam ahead” 30 Scenario B: “Knowing the ropes” 32 Scenario C: “Sink or swim” 34 Scenario D: “In the doldrums” 36 Chapter 3: Technology investment 38 Technology investment 40 – Historical uptake of new technologies – Ship owner survey Chapter 4: Methodology and assumptions 46 Methodology and assumptions 48 – Simulation model – Assumptions Chapter 5: Technology uptake in Shipping 2012–2020 52 Technology uptake in shipping 2012–2020 54 – Results – Ballast water – SOX – NOX – CO2 and energy efficiency – Fuel mix and demand – Sensitivity analysis Chapter 6: Trends and drivers beyond 2020 62 Trends and drivers beyond 2020 64 – Fuel – Regulations – Innovation and adoption of new solutions ©Getty Images shipping 2020: 03 Executive summary Based on trends in the world economy and transport demand, marine regulations and technology, we have described four possible development paths as we move towards 2020, Scenarios A to D. These scenarios give input to a simulation model developed to assess likely technology investment in the world fleet from 2012 to 2020. This project has used a scenario approach to assist to describe likely outcomes on technology uptake in the maritime industry. The four scenarios A–D each provide a different picture of how the shipping fleet would appear in 2020. The scenarios are described in details later in this report, but to better appreciate the main findings, as described below, the scenarios can be summarized as follows: Scenario A (“Full steam ahead”): High economic growth; high fuel prices; little regulatory or stakeholder pressure on the environment Scenario B (“Knowing the ropes”): High economic growth; LNG prices low and decoupled from oil prices; high regulatory and stakeholder pressure on the environment need for low sulphur fuel. Even given a large number of scrubbers, the major part of the fleet will run on distillates. DNV estimates that the demand for LNG will be 8-33 million tonnes in 2020, depending on the scenario selected, the equivalent of 400 to 1,700 MMBtu. The consumption of HFO will, according to our simulations, drop from approximately 290 million tonnes in 2019 to only 80-110 million tonnes in 2020, unless the global sulphur limit is delayed, in which case HFO demand will be over 300 million tonnes annually. Scenario C (“Sink or swim”): Low economic growth; low fuel prices in general but high demand keeps the marine gas oil (MGO) prices up; high regulatory and stakeholder pressure on the environment A No ships on destillates CDB 40,000 ships on destillates Scenario D (“In the doldrums”): Low economic growth; LNG prices decoupled from oil prices; low regulatory or stakeholder pressure on the environment. This study has identified key findings concerning the main regulatory issues, highlighting important considerations for ship owners and operators in the period leading up to 2020. The key findings from the simulation model are summarized here. Finding 1: More than 1 in 10 newbuildings in the next 8 years will be delivered with gas fuelled engines. The number of liquefied natural gas (LNG) fuelled ships through 2020 depends heavily on fuel prices. With a LNG price 10% above heavy fuel oil (HFO), 7-8% of newbuildings from 2012 to 2020 will be able to run on LNG. If the LNG price goes down to 30% below HFO, the uptake of LNG increases to 13% and, in the extreme case of LNG price 70% below HFO, the LNG share of newbuildings is 30 percent. In total numbers, 13% is equivalent to approximately 1,000 ships. The global sulphur limit, if effective beginning in 2020, combined with the 20% EEDI reduction requirement, will have a significant impact on the implementation of gas fuelled engines provided the capacity and fuel sup- ply are there. We could see up to half of the newbuildings being delivered with gas fuelled engines by 2020. CAD No LNG fuelled ships B 5,000 LNG fuelled ships Finding 2: In 2020, the demand for marine distillates could be as high as 200-250 million tonnes annually. The current global demand for marine distillates is approximately 30 million tonnes annually. The 0.1% limit in Emission Control Areas (ECA) will increase the demand to approximately 45-50 million tonnes by 2015. However, the largest increase will be experienced in 2020, with the introduction of the global sulphur limit. This marks a huge increase in the need for distillates, an increase of up to 200-250 million tonnes. In the short term, the use of LNG and scrubbers will have only a limited impact on the Finding 3: Newbuildings in 2020 will emit up to 10 to 35% less CO2 than today’s ships, and the Energy Efficiency Design Index (EEDI) will be a driver for more than half of the reduction. Environmentally efficient designs will gradually improve throughout this decade and a newbuilding contracted in 2020 will, depending on type, emit 10-35% less CO2 than a current ship with an EEDI equal to the IMO reference line. The largest reduction will be experienced with tank, bulk and container vessels. Between one-third and one-half of this reduction will be motivated by cost-efficiency alone and would be implemented regardless of the EEDI requirements. EEDI will be an important driver for the remaining reductions in particular from 2020 forward when Phase 2 of the EEDI is implemented, requiring new ships to be 20% below IMO reference lines. A No scrubbers BD C 20,000 scrubbers 04: shipping 2020 Finding 4: Scrubbers are a significant option after 2020. When the global sulphur limit is enforced in 2020, ships would be required to run on low sulphur fuel or clean the exhaust continuously. This will have a significant impact on the implementation of scrubbers. This technology may potentially be fitted to thousands of ships if there is availability and the capacity to deliver. In Scenario C, with high LNG price and MGO price, the number of scrubbers in 2020 is expected to be nearly 20,000, while in Scenarios B and D, 13-14,000 would be expected. not enter into force, a significant number of installations of treatment systems is still expected. The main peak is expected in 2017, when the last ships with medium ballast water capacity have to retrofit at the same time as the rest of the fleet has to start retrofitting. After 2019, the retrofitting is expected to be largely completed. C A B D No BW treatment BW treatment for all ships The full effect of the regulatory requirements on technology uptake will come after 2020, when the IMO global sulphur limit and Phase 2 of the EEDI are implemented. These are by far the strongest drivers and may lead to fundamental changes in the shipping industry. The uncertainty of whether the limit will be enforced in 2020 or in 2025 may delay the use of scrubbers, as ship owners would not be expected to take on the additional cost without this being resolved. In Scenario A, the global sulphur limit will not come before 2025 and the number of scrubbers in place in 2020 is very limited. Even though there may be a significant uptake of scrubbers from 2020, in Scenario C with the highest LNG and MGO price, which is favourable for scrubbers, there would only be a modest 50 installations annually up to 2019. DC A B Finding 6: At least 30-40% of newbuildings will be fitted with EGR or SCR by 2016. The Tier III 80% reduction requirement in ECAs is the main challenge for NOx. In Scenarios A and C, approximately 40% of ships built between 2016 and 2020 will have implemented an exhaust gas recirculation (EGR) or selective catalytic reactors (SCR) system, while in Scenarios B and D, the numbers are approximately 30% and 35% respectively. The difference in fuel prices seems to account for this difference. When the price of LNG is low compared to MGO, NOx requirements are met with LNG fuelled engines. 3,000 ships >10% CO2 reduction 8,000 ships >10% CO2 reduction Finding 5: Ballast water treatment systems will be installed on at least half of the world fleet. The Ballast Water Management Convention (BWMC) has not yet entered into force, but the schedule for mandatory treatment of ballast water is fixed independent of when the convention is ratified. In addition, the US has decided to implement a similar scheme for all ships in US waters, which would force a significant part of the world fleet to implement a treatment system irrespective of BWMC progress. For the two Scenarios A and B, which assume the ballast convention does Without a Tier III compliant engine, a ship built after 2016 will not be able to enter the two existing ECAs. The question that remains to be answered is whether a ship owner will opt for a Tier III engine even if the ship is initially not planned for sailing in an ECA, as the second-hand value of the ship might be lower due to the loss of geographic flexibility. DC BA No ships with EGR or SCR 2,500 ships with EGR or SCR shipping 2020: 05 Introduction Shipping 2020 – a report on technology uptake for the maritime shipping industry. When travelling around the globe, I have repeatedly been asked the same question: Which technologies, known or unknown, do you think will be adopted by the shipping industry in the years to come? With the world economy in a fragile condition, it is not only financial analysts, bankers and policy makers who would like to know what the world will look like towards the end of this decade. The shipping community is, rightly, asking the same questions; Which drivers will influence technology choices in the years to come? Which technologies are most likely to be adopted by the industry? In other words, based on projections and likely development scenarios, and from a technology perspective, what will the world fleet look like in 2020? Our objective has been to share our views on technology uptake towards 2020 and beyond, and to stimulate discussions about likely options for the industry. Predicting the future is a risky business. However, in this report we have done just that. Our objective has been to share our views on technology uptake towards 2020 and beyond, and to stimulate discussions about likely options for the industry. We will adress questions such as how we think environmental regulations will influence the use of NOx, SOx, Ballast Water (BW) and Greenhouse Gas (GHG) related technologies in the future. Will scrubbers dominate, or will there be a significant switch to Liquefied Natural Gas (LNG) as fuel? What will the speed of implementation be? Needless to say, there are no precise answers to the questions discussed in this report. That said, we consider DNV to be very well positioned to identify and discuss future technology trends. Our voice is grounded in the expertise and skills of our truly global work force. Furthermore, we enjoy close working relationships with ship owners, operators, yards and suppliers from all over the world. We also work actively with international forums, such as the IMO, flag states and many other organisations. Each year we run hundreds of joint industry projects and other technology qualification projects with the maritime community, and we invest close to 6% of our revenue in research & innovation activities. Perhaps most importantly, DNV is an independent foundation with no stakes in the commercial fate of any technology that may be a preferred choice in the future. Regardless of future technology choices, our purpose is, and will always be, to “safeguard life, property and the environment”. Part of this purpose is also thus to be the leading firm in terms of technology. In the final analysis, the results hinge on all of the assumptions made, some of which are well documented and acknowledged while others are more contentious or difficult to quantify. We have based our scenarios and assumptions on comprehensive literature research, questionnaires, external forecasts and, finally, ‘guesstimates’ where necessary. Thus, even though this is not a scientific report in which everything has been meticulously quantified, we are confident that the results presented provide a solid guide as to what we can expect in the years to come. © DNV/Nina Rangøy The experienced project team behind this report represents all aspects of international shipping: commercial, technical, regulatory, environmental and modelling/simulation experts. The core project team has consisted of Ole Vidar Nilsen, Tore Longva, Christer Farstad, Magne Berg, Toril Bendiksvoll, Leif Braute, Serge Schwalenstocker and Jannicke Witsø plus important contribution from many other DNV people. Thanks also to Coor Media which has been responsible for the design of the final product. We hope we have communicated our enthusiasm for the subject in this report, and we do hope you will enjoy the following pages – happy reading! Tor E. Svensen president, dnv Maritime and Oil & Gas 06: shipping 2020 shipping 2020: 07 ©© GDeNttVy/INmiangaesE.Rangøy 08: shipping 2020 Chapter 1: Trends and drivers Shipping is a complex and volatile industry that is constantly navigating the many twists and turns of the global economy. While currently going through a tough time, the industry is cyclical by nature and has experienced many peaks and troughs in the past. Given the complexity that characterises the shipping industry, how can one predict what technologies will be implemented in the world fleet by 2020? In this chapter, we look at four important driving forces and trends that we believe will impact future technology ‘uptake’ and the development of the world fleet as we move towards 2020. The first part of the chapter outlines the economic factors that may affect the demand for and supply of vessels. The second part of the chapter takes a closer look at the regulatory outlook, while the third part discusses various technologies as solutions to the possible future regulatory landscape. In the final part of the chapter, we take a closer look at fuel trends and possible development in the prices of various types of fuel. © Valderhaug INDEX •••• The world economy and demand for seaborne transport 10 •••• Environmental regulations 16 •••• Technology and energy efficiency 20 •••• Fuel trends 24 shipping 2020: 09 •••• The world economy and demand for seaborne transport The world economy is highly dependent on shipping, which meets approximately 85% of the global demand for transport. Over time, changes in global growth are expected to lead to changes in the world’s seaborne trade centre of gravity. The shipping market is characterized to a great degree by market cycles. This volatility creates a potential for high profits, but also for considerable losses. With the current reduced level of world economic growth coinciding with overcapacity in the global fleet, the shipping market is experiencing tough conditions. The next few years will be critical for shipping and for the balance between supply and demand. Three economic areas currently define the maritime trade: North America, Europe and Asia. The shift in the relative importance of these areas will affect the maritime industry substantially in the years to come. During the past decades, the world economy has demonstrated stable growth, occasionally interrupted by recessions of different magnitudes. In 2009-2010, the world economy was in its weakest state since the Great Depression of the 1930s, but it has been slowly recovering since. As can be seen in the figure below, world economic growth and the demand for seaborne transport are closely linked. A general observation is that during periods of low world Gross Domestic Product (GDP) growth, the volume of seaborne trade shrinks. Thereafter, the world situation changed; the canal was reopened and US oil imports shrank, resulting in lower than expected overall demand. This led to a weak shipping market during the 1980s. The poor market conditions caused bankruptcies, distress sales of tonnage and higher scrapping rates. The situation started to improve during the 1990s. Since 1995, there have been relatively large variations in the growth of the world fleet and seaborne trade. The relationship between the demand for shipping and the capacity of the fleet was quite balanced during the 1960s. This was followed by a period of heavy investment in shipping during the 1970s, with increasing US imports and the closing of the Suez Canal. Regional variations Changes in merchant ship trade patterns have traditionally followed regional variations in world growth. In the 1970s, the container trade was dominated by Japan, whereas, since 2000, China’s annual container trade has grown by an average of 30 percent. Today, China is by far the largest container market. China’s economic growth started in the 1990s. During the decade between 1998 and 2008, China added 1 billion tonnes of seaborne cargo and represented 60% of world growth in seaborne trade. The bulk carrier shipping boom was driven by Chinese steel production, which peaked at close to 50 million tonnes per month in 2008. An immediate demand for shipping resulted in the rapid contracting of new vessels. Squeezed shipyard capacity led to substantial growth in newbuilding prices. For example, new Capesize bulk prices increased from $35-40 million in 2003 to $95-100 million in 2008. In addition, in 2008-2009, the world shipping order book was close to 50% of the existing world fleet. From 2002, globalisation and the rapid development of Asian economies have led to a great deal of added demand in the shipping market. This resulted in unprecedented contracting of new tonnage, boosting the order book by as much as 500 percent. The financial crisis in 2008 led to world trade shrinking dramatically. Naturally, this created a substantial oversupply of tonnage in the market which inevitably led to depressed freight rates and weak markets in general. % growth year on year 1966 1968 1970 1972 1974 1976 1978 1980 1982 1984 1986 1988 1990 1992 1994 1996 1998 2000 2002 2004 2006 2008 2010 2012 2014 14% 12% 10% 8% 6% 4% 2% 0% -2% -4% -6% -8% -10% Seaborne trade (left-hand axis) World GDP (right-hand axis) Figure 1: The relationship between GDP growth and seaborne trade growth. Source: Clarkson Research Services Ltd. 10: shipping 2020 7% 9% 6% 7% 5% 5% 4% 3% 3% 1% 2% -1% 1995 1997 1999 2001 2003 2005 2007 2009 2011 2013 2015 2017 2019 1% -3% 0% -5% -1% -7% Total fleet Seaborne trade Figure 2: Growth in the world fleet and total seaborne trade (forecast included). Source: IHS Global Insight and SAI data as of February 2012. Despite the uncertainty tied to developments in the global economy, there are some bright spots in both the country and industry outlooks. growth trends in commodities are presumed to continue. 2015 4.8 4.2 1.3 0.6 2010 3.4 3.1 1.0 0.4 0 1 2 3 4 Dry Bulk Liquid Bulk 5 6 Container 7 8 9 General Cargo Billion tonnes 10 11 12 Figure 3: Seaborne trade by commodity (forecast included). Data based upon IHS Global Insight as of July 2011. Source: IHS Global Insight and SAI data as of February 2012. The capacity of the world fleet is expected to grow during the next ten years. IHS Fairplay estimates that the total fleet, measured in million dwt, will increase by approximately 50% between now and 2020, based on annual economic growth of 3.3 percent. This suggests that an average of 1,700 to 2,000 new vessels will be contracted for in each year. Implications The shipping market is currently in a trough. The vessels that were ordered during the market boom are now being delivered, adding to the difficulty of adjusting to today’s less favourable economic situation. It will take some years before the current oversupply is absorbed. As the BRICKTIM countries are expected to World GDP growth was estimated to shrink by offer even more competitive prices as they have higher growth rates than the Western 4.2% in 2010 and by 3% in 2011, and be gradually run out of orders. Newbuilding world, it is assumed that the centre of gravity approximately 2.7% in 2012. China’s econ- prices have already fallen on average by more of economic power will continue to shift. omy has shown a lot of resilience and repre- than 40% compared to 2008. Trade patterns change and consequently the Billion tonss2en0t1s080% of the GDP in East Asia and Bthilelion tons 2015 Pacific region, but its growth rate is expected Outlook relative weights of shipping trade routes are expected to do the same, increasing the to decrease to 7.8% in 2012. The Eurozone Looking ahead, we are in for a period of power of the emerging economies at the 1,0 0,4 outlook is uncertain due to the sovereign 0,6 change in world economic power. The centre debt cr3is,i4s, as are its potential negative1r,e3per- of economic activity will shift toward Asia. US expense of the OECD countries. cussion effects on the global economy. fiscal able. Tchhae4lrlee,8nagreesbarrigehetxppreocstpedecttos beDmryaBnauglke-s within some The changes in the world economy and global seaborne transport described above Despite the uncertainty tied to developments industries, especially the energy seLctiqoru. iTdhBeulk provide the premises for how the various ship in the global economy, there are some bright UK and Germany have shown positive signs segments will evolve. In the next sections, we 3,1 spots in both the country and industry 4ou,2t- of recovery; however, recent data sCugognetsat itnheatrs will take a closer look at the resulting trends looks. Global seaborne trade has recovered both economies are flattening out. The sover- in the main ship segments covered in the from the recession in 2010, increasing by eign debt crisis in Europe is still in tGheenbaelraanlcCe.argroe/pNoertr.o Bulk 8.6% in 2010 and 7.4% in 2011, and is antici- Brazil, Russia, India, China, Korea, Turkey, pated to grow another 8.5% in 2012. Indonesia and Mexico (BRICKTIM) still have strong development potential and their GDP Despite relatively strong growth in seaborne per capita figures are expected to increase trade, supply is still overwhelming. Hence, no substantially, by approximately 30% between major market improvements are expected in now and the year, 2015. It is assumed that by the short term. The new vessels ordered in 2020, 40% of world economic activity will take 2011 accounted for 72 million dead weight place in Asia. The total world GDP growth is tonnes (dwt), down from 151 million dwt in expected to remain fairly stable at approxi- 2010, and this figure is expected to remain mately 3.3% annually through 2030. The total low. A shrinking order book will increase the seaborne trade is anticipated to grow by an pressure on yards, which will be forced to average of 6% year-on-year towards 2016. The shipping 2020: 11 Trends in the tanker market The crude oil tanker market is currently suffering from a very depressed situation with an abundance of tonnage. Owner groups have been losing money for a long time and some companies are on the brink of bankruptcy. Significant deliveries of crude tankers in 2012 may depress rates further. Scrapping can only partly ease the challenges in this market. The growth in demand will predominantly come from non-OECD countries. The product tanker market is also struggling with oversupply and is subject to continuous changes in import needs and trade patterns. The reduction in trans-Atlantic trade due to lower consumption is noticeable. However, while the current order book for product tankers is limited, some owners believe this market will pick up sooner than the crude tanker market. The demand for LNG is on the rise, new LNG plants are being built in many countries and LNG carriers are enjoying very high rates due to the surge in consumption, resulting in a positive outlook for the LNG ship segment. The LNG market is dependent on developments in the gas price and on environmental pressure to use cleaner fossil fuel. We are likely to see continued growth in demand in this market, especially from 2015-2020, and even more so beyond 2020. Based on this, we expect a rapid expansion of LNG bunkering networks. By 2020, oil imports to China and India are likely to have increased compared to 2012 levels. The supply will come primarily from the Middle East, Africa and Russia, and also by pipeline. Substantially higher oil production levels from offshore Brazil are expected. Currently, Brazil’s offshore production is 2 million barrels per day (mbd). This is expected to increase to 4 mbd by 2017 and 5 mbd by 2020. Meanwhile, US crude imports will focus on shorter hauls, mainly from South America. 125.0 22.8 63.5 36.8 US Canada Mexico South and Central America Europe and Eurasia Middle East Africa Asia Pacific 295.2 33.9 33.3 36.9 83.8 45.7 28.9 86.0 109.3 116.7 83.0 21.3 43.7 24.1 179.9 118.4 45.4 129.6 28.6 227.1 28.8 39.5 37.6 20.0 Tanker owners have experienced strong pressure from oil majors for many years, as well as vetting schemes with respect to the quality and integrity of vessels, and this trend is expected to continue. In part, this is due to the fact that oil companies generally have very high technical and operational standards for oil tankers. Additionally, as bunker prices remain high, the unit transport cost is a driver for fuel efficiency, as in all shipping markets. Provided the scrapping rate picks up and demand for crude tanker capacity follows the growth in Chinese demand and in the number of oil supply sources, we may expect the market to be in balance well before 2020. Figure 4: Major oil trade movements in 2010. Source: BP Statistical review of World Energy 2010. % growth year on year 1995 1996 1997 1998 1999 2000 2001 2002 2003 2004 2005 2006 2007 2008 2009 2010 2011 2012 2013 2014 2015 2016 2017 2018 2019 2020 10% 8% 6% 4% 2% 0% -2% -4% -6% -8% -10% Crude oil tanker fleet Crude seaborne trade 12: shipping 2020 Figure 5: Annual growth in the crude oil tanker fleet and crude oil seaborne trade (forecast included). Source: IHS Global Insight and SAI data as of February 2012. Trends in the dry bulk market The dominant factors for the dry bulk market are commodity supply industries such as energy, manufacturing and construction. As we have discussed previously, world trading activity is closely correlated to global GDP levels. In the past, the economies of the US, Europe and Japan have acted as the primary drivers of the dry bulk trade market. However, the contributions of China, India and Brazil are becoming more important as these countries’ GDP growth rates are significantly higher than those of the developed countries. China is by far the largest contributor, and has been so for the past five years. Iron ore seaborne trade routes in 2010 (in mill. tons) World trade: 1,3 bill. tons Despite this, time charter rates in the whole market are significantly down since the 20032008 period. The continuous supply of new ships throughout 2012 and 2013 due to the large order book is a significant contributor to the prolonged deflated market, and the outlook towards 2020 remains challenging. Australia and Brazil are the world’s largest iron ore exporters and China is by far the largest buyer. This pattern is expected to continue as global steel demand is set to increase significantly as we approach 2020 and beyond. China has surpassed Japan as the world’s largest importer of coal. Analysts believe Total exports Iron ore seaborne trade routes in 2010 (in mill. tons) World trade: 1,3 bill. tons Total imports Total exports Total imports Russia 33 Russia 33 38 38 Canada Canada 17 17 North America North America Iron ore seaborne trade routes in 2010 (in mill. tons) World trade: 1,3 bill. tons Total exports Total imports Brazil 344 Colombia 59 Coal seaborne trade routes in 2010 (in mill. tons) World trade: 909 mill. tons 115 122 Europe 115 122 849 218 Europe China 27 133 171 Japan 849 218 47 116 KoCrehaina 27 Brazil 344 106 67 South Africa Colombia 59 145 104 India 145 104 268 India Indonesia Australia 106 67 459 297 South Africa Russia 33 268 Indonesia Australia 459 297 133 171 Japan 47 116 Korea Total exports Total impor3t8s Coal seaborne trade routes in 2010 (in mill. tons) Canada World trade: 909 mill. tons 17 115 122 Europe 849 218 China 27 133 171 Japan 47 116 Korea TotaNloertxhpAomretsrica Figure 6To:taIrl oimnpoortrse and coal seaborne trade route14s5 in1042010 India (in mill. tonnes). Source: IHS Global Insight. Brazil 268 344 Colombia 59 106 67 South Africa Indonesia Australia 459 297 that the high volume of imports will remain strong and that an increase in coal demand will give the bulk fleet a boost. Furthermore, it is expected that India’s iron ore exports will fall in order to protect national steel production. This suggests that China will need to source vessels from more distant parts of the world. India will, however, need to import large amounts of coal in the coming years due to the investments made in several large power projects. All in all, it is expected that the trade between Africa-Asia and the Middle East-Asia will keep growing at a higher pace than the rest of the world as we approach 2020, and this will have a positive effect on the dry bulk market. The composition of the dry bulk fleet in 2020 is expected to be influenced by the factors described above. With respect to both supply and demand, we will see stronger growth below 100 thousand dwt and above 200 thousand dwt. All in all, analysts believe we will see sharp growth in the bulker fleet in 2012 and 2013, although thereafter, the dry bulk fleet is expected to grow by less than 5% per year up to 2020. Vessel values and earnings will continue to be under pressure for years to come as a result of the current oversupply. % growth year on year 1995 1996 1997 1998 1999 2000 2001 2002 2003 2004 2005 2006 2007 2008 2009 2010 2011 2012 2013 2014 2015 2016 2017 2018 2019 2020 Coal seaborne trade routes in 2010 (in mill. tons) World trade: 909 mill. tons T1o8tal exports T1o6tal imports 14 12 10 8 6 4 2 0 -2 Bulker fleet Dry bulk trade Figure 7: Annual growth in the bulker fleet and dry bulk seaborne trade. Source: IHS Global Insight and SAI data as of February 2012. shipping 2020: 13 Trends in the container market This market is “the closest to the consumer” from reefers and roll-on/roll-off vessels. The are growing at an annual rate of 10% or and, by its nature, the demand is strongly container feeder vessel market is experienc- more. The growth in Inter-Asia and Inter- driven by the GDP developments in the ing competition in parts of the world, in China trade is even stronger. world, demographic developments and, not Europe, in particular, and is losing out to the least, changes in per capita income in regions direct service offers of larger container vessels China is currently building a self-sustaining and large countries. Underpinning this trade with lower bunkering costs per unit. supply chain between itself and developing es is the development of goods containerisation. We have seen tremendous developments in Container business developments are influ- nation trade partners in order to protect its supply and investments abroad. This will this area over the past 30 years. enced by ongoing changes in trade patterns, bring both structural changes to the con- some of which can be regarded as emerging tainer (and dry bulk) industries and the The current situation is not very positive, with long-term trends. We see a slowdown in the demand for vessels. It is likely that this is the an imbalance between supply and demand. growth of the mainline trades, Asia-North trend that will accelerate most quickly over Ships on order represent approximately 25% America and Asia-Europe, and an increase in the next decade. of the existing container fleet. The situation the Europe-Middle East, Middle East-Asia and is more positive for general cargo, as that South America-Africa-Asia trade routes. The unit transport cost is a driver for fuel effi- fleet is shrinking somewhat in size. Container Mainline trades are growing at approximately ciency and larger vessels. Infrastructure devel- vessels are furthermore taking market shares 5% per annum whereas non-mainline trades opments to support larger vessels in this trade will continue and will reinforce this trend. Latin America Africa Indian sub. Cont. Australasia Figure 8: Container trade routes, main and non-mainline trades. Source: Dynamar. 18% 16% 14% 12% 10% 8% 6% 4% 2% 0% -2% -4% -6% -8% -10% Container seaborne trade Container fleet 1995 1996 1997 1998 1999 2000 2001 2002 2003 2004 2005 2006 2007 2008 2009 2010 2011 2012 2013 2014 2015 2016 2017 2018 2019 2020 Figure 9: Annual growth in the container fleet and container seaborne trade (forecast included). Source: IHS Global Insight and SAI data, February 2012. Analysts believe that the composition of the container carrier fleet in 2020 will be influenced by the above trends. The large ships of 14,000 twenty-foot equivalent units (teu) and above will serve the main trade lines such as Asia-Europe. The Asia-American West Coast trade will also deploy larger vessels, up to the new Panamax standard of 12,500 teu. The rapid growth of ship size beyond the 18,000 teu mark may continue, but a practical maximum ship size limit of more than 20,000 teu is likely to be established in the next decade. For the emerging markets and inter-Asia and inter-China trades, we will see an increase in the number of 4,000-8,000 teu vessels, while vessels smaller than 1,000 teu are likely to represent a smaller share of the market in 2020 than they do today. The liner companies’ consolidation efforts may also succeed. If so, the top ten liners in 2012 may be reduced to 4-5 companies, each covering a larger share of the global box trade in 2020. In comparison, the car carrier segment, which is also “close to the consumer,” is thriving on the world’s need for cars. In this segment, we expect new orders to keep up with growing demand. 14: shipping 2020 Trends in the offshore market This market is driven by the high price of oil and by investments in offshore exploration and production (E&P). These drivers apply consistently, with relatively low growth in the world. Existing resources on land and in shallow waters are being depleted, and new discoveries are being made in deeper and harsher environments, such as in offshore Brazil, West and East Africa, the Arctic region and Southeast Asia. As the world will need fossil fuels at an increasing rate for the foreseeable future, overall offshore expenditures will increase in the next 10-20 years, and are expected to grow between 10-15% on average per annum, This segment is also affected by new demand for specialised vessels intended for use in, for example, offshore wind developments. As earnings and profits activities continue to move into new areas and deeper and harsher environments, the demand for specialised offshore vessels will grow further. Few vessels built before 1995 are tailored for deeper subsea support and offshore construction support, indicating a relative shift towards deep and ultra-deep support capability. In particular, we will see an increase in the demand for larger offshore ships in general and for high specification and more advanced ships to support safe operations in harsh environments. Today, offshore support vessels are the fastest growing ship type within the offshore segment, and we expect this to continue as we move toward 2015-2020. Strong global pressure and attention to environmental protection of pristine areas, as well as any binding agreements on cuts in greenhouse gas emissions, may increase the demand in this shipping market and accelerate the technology uptake. The offshore segment is leading in employing new and innovative technology. North America 1018 243 712 NW Europe 627 273 The Mediterranean 515 160 Middle East 183 Active fields and fields under construction Potential or prospect fields 390 West Africa 222 403 Latin America 192 Figure 10: Status of offshore field developments. Source: Clarkson. 717 Asia Pacific 726 2020 455 5659 1,134 4,652 3,034 2012 384 4,117 964 3,734 2,159 0 800 1,600 2,400 3,200 4,000 4,800 5,600 6,400 7,200 8,000 8,800 Number of ships Figure 11: Development of the offshore fleet (forecast included). Source: SAI data, February 2012. Crew/supply vessel Platform supply ship Offshore tug/supply ship Anchor handling/tug supply Support/safety shipping 2020: 15 •••• Environmental regulations International shipping is a heavily regulated industry. In this decade, it will see a plethora of additional regulations becoming effective, with significant economic and operational implications. Managing their cumulative impact may be one of the decade’s key challenges for individual companies; those not making the right strategic choices may face a severe impact on their long-term viability. Key environmental regulations coming into force in this decade address emissions of sulphur oxides (SOx), nitrous oxides (NOx), particulate matter (PM) and greenhouse gases (in particular CO2), as well as ballast water management. New international regulations addressing ships’ energy efficiency enter into force on January 1, 2013, while stricter sulphur requirements enter into force for specific sea areas in 2015 and globally in 2020, and demanding ballast water treatment requirements are expected to enter into force before the middle of the decade. Compliance is made challenging by a number of factors, including financial constraints, technological immaturity and uncertainty regarding enforcement and the consequences of non-compliance. The cost of compliance will be high for the maritime industry, and the business consequences of wrong decisions severe. In the longer run, the ability to navigate these regulatory waters is likely to be a key commercial differentiator. Environmental regulations on the agenda Shipping currently operates under a complex set of international and national regulations. Traditionally, the leaps in regulations have been event driven, and in some cases even driven by events outside the sector. A wellknown example is the Exxon Valdez oil spill, which resulted in the US Oil Pollution Act (OPA) and mandatory double hull design. While environmental regulations in the maritime industry have historically lagged behind those of other industries, this situation is now changing. An increased focus on both global and local environmental issues in general, combined with the growing realisation of the actual pollution burden imposed by shipping, has led to an upsurge in both international and national regulations. Some are ready for implementation and will enter into force in the near future, while others are still being developed and will have an impact only in the intermediate term. The key issues having a significant regulatory impact this decade are, broadly speaking, SOx, NOx, particles, greenhouse gases (in particular CO2) and ballast water management. From a “beyond 2020” perspective, there are a number of emerging issues that appear likely to result in regulatory initiatives: key among these are black carbon, hull bio-fouling and underwater noise. Sulphur oxides, nitrous oxides and particulate matter SOx, NOx and PM are all emissions to air that result from the combustion of marine fuels. These emissions have potentially severe ecosystem impacts and negative health effects on exposed populations. These impacts have, in some parts of the world, led to strict regulation of emissions from land-based sources. In recognition of shipping becoming a dominant emission source, potentially exceeding landbased sources, emissions have been internationally regulated by the IMO. MARPOL Annex VI states a combination of general maximum global emission levels and more stringent levels applying to designated sea areas generally known as Emission Control Areas (ECAs). The regulations allow emissions to be mitigated by either changing the fuel 2010 2011 2012 2013 2014 1.0% ECA sulphur limit 3.5% global sulphur limit NOx tier II for newbuild- ings HFO prohibited in Antarctica 0.1% sulphur limit in California BW convention entry into force (?) North American ECA Entry into force of EEDI and SEEMP 2010 2011 2012 2013 2014 Figure 12: Upcoming maritime regulations. Source: DNV. 16: shipping 2020 2015 2016 2017 2018 2019 2020 0.1% ECA sulphur limit EU MBM fully Implemented (?) NOx tier III for newbuild- ings IMO low sulphur availability review 0.5% global sulphur limit (delay until 2025?) Entry into force of recycling convention (?) BW treatment on all ships (?) 2015 2016 2017 2018 2019 2020 The ability to navigate these regulatory waters is likely to be a key commercial differentiator. specification/type or by exhaust gas cleaning. By 2015, operators will have to choose between installing exhaust gas cleaning systems known as scrubbers or switching to low sulphur fuel for all ships operating in an ECA. In 2020 or 2025, pending an IMO decision in 2018, the 0.5% sulphur global cap will enter into force. A complicating factor in these decisions is the South of 62 N fact that there are local and regional regulatory initiatives in addition to the international East of 4 W IMO requirements. One key example is the EU, where the most likely outcome of an 57.44.08 N Baltic Sea ongoing revision of legislation is the implementation of a 0.5% sulphur limit in all EU Atlantic Ocean North Sea waters, beginning in 2020. These develop- ments may significantly affect operator considerations. East of 5 W Uncertainties notwithstanding, international regulatory deadlines are clear and key strategic decisions need to be made. Ballast water Another key issue likely to have a significant regulatory impact this decade is the implementation of the new IMO Ballast Water Convention. There have been many cases of alien species being introduced into new environments, with ballast water being a major transfer mechanism in the world today. Organisms carried with ballast water can establish themselves in new environments, causing dramatic shifts in food webs, chemical cycling, disease outbreaks and indigenous species extinction rates. The cost of these invasions has been estimated to be in excess of $8 billion annually in the US alone. In response to this, the IMO adopted the Ballast Water Management Convention, a set of regulations which severely limits the amount of organisms carried in ships’ ballast water. A key provision of the convention makes ballast water treatment mandatory for all ships in accordance with a defined schedule. The convention is unique in that it has a fixed timeline, mandating that most ships in international trade have to have ballast water treatment systems installed by the end of this decade. Pacific Ocean Figure 13: Designated Emission Control Areas (ECA). Source: The IMO. Atlantic Ocean shipping 2020: 17 Figure 14: DNV concept ship “Triality” is designed to comply with future environmental regulations. New cargo tank divisions eliminate the need for ballast, including during cargo operations. Source: DNV. Size Phase 0 Phase 1 Phase 2 Phase 3 1 Jan 2013– 1 Jan 2015– 1 Jan 2020– 1 Jan 2025 31 Dec 2014 31 Dec 2019 31 Dec 2024 onwards Bulk carriers >20,000 Dwt 0% 10–20,000 Dwt n/a 10% 0–10%* 20% 0–20%* 30% 0–30%* Gas tankers >10,000 Dwt 0% 2–10,000 Dwt n/a 10% 0–10%* 20% 0–20%* 30% 0–30%* Tanker and combination carriers >20,000 Dwt 0% 4–20,000 Dwt n/a 10% 0–10%* 20% 0–20%* 30% 0–30%* Container ships >15,000 Dwt 0% 10–15,000 Dwt n/a 10% 0–10%* 20% 0–20%* 30% 0–30%* General cargo ships >15,000 Dwt 0% 3–15,000 Dwt n/a 10% 0–10%* 15% 0–15%* 30% 0–30%* Refrigerated cargo ships >5,000 Dwt 0% 3–5,000 Dwt n/a 10% 0–10%* 15% 0–15%* 30% 0–30%* Figure 15: Reduction factors (in percentage) for the EEDI relative to the reference line for each ship type. Source: IMO, MARPOL Annex VI. The convention is close to its ratification threshold, and once ratified, a strong surge in system demand can be expected. There are numerous suppliers in the market providing IMO-approved ballast water treatment systems’ that utilise different physical processes. All systems, however, have relatively limited operational experience and come with an inherent technology uncertainty. Furthermore, as all systems’ performance is dependent on water quality, trading pattern specifics may be a crucial determinant when choosing a technology. Regarding ballast water management, the US will not be ratifying the IMO convention in the foreseeable future. It has instead pub- 18: shipping 2020 lished its own federal legislation with a discharge standard identical to the IMO requirements and an implementation schedule close to that of the IMO. However, a key difference from the IMO regulation is the equipment approval scheme which makes it, at this date, uncertain whether equipment approved under the IMO scheme will be able to meet US requirements. Based on the fact that the Ballast Water Management Convention has not yet entered into force, and that the price for ballast water treatment systems has run into several million US dollars per ship, industry uptake has been slow. However, the effective result of the ratification threshold being reached in the near future will mean that several thousand ships will need to have such systems installed within a very short time span. Further delays in ratification are expected to exacerbate the situation. It remains an open question whether the supplier, yard and engineering capacity will be sufficient to meet the surge in demand. Greenhouse gases Greenhouse gases such as CO2 are the primary mechanism for anthropogenic warming of the atmosphere, with the international community working for more than 20 years to establish effective international regulations. With the resurgence in international concern regarding CO2 emissions in 2005 and 2006, IMO committed itself to addressing ships’ CO2 emissions through a combination of technical, operational and market-based measures. This commitment was stimulated by the European Council decision to develop regional CO2 control mechanisms if effective international mechanisms were not in place by the end of 2011. Protracted negotiations at the IMO led to the adoption of the Energy Efficiency Design Index (EEDI) and Ship Energy Efficiency Management Plan (SEEMP) in 2011. These enter into force effective in January, 2013. By setting increasingly stringent energy efficiency requirements for new ships, the EEDI is intended to stimulate the development of more energy efficient ship designs, thereby indirectly leading to reduced operational CO2 emissions. The SEEMP, on the other hand, is designed to directly stimulate more energy efficient operational practices. While these IMO regulations are recognised as significant achievements by the international community, work done jointly by DNV and Lloyd’s Register nevertheless indicates that the impact will only be a lower emission growth rate, not an absolute decrease. The political pressure on the IMO to achieve real reductions has therefore not diminished. The mechanism remaining on the table at the IMO, Market Based Measures (MBMs), has been highlighted as potentially capable of delivering real reductions. Negotiations on MBMs have nevertheless met with significantly less success than those on the EEDI and SEEMP. The reasons are multifaceted, but broadly speaking boil down to fundamental disagreement between developing countries and the developed world on the applicability of such mechanisms. Until this issue is resolved, there is limited likelihood of the IMO progressing on the issue. 18,000 16,000 14,000 12,000 10,000 8,000 6,000 4,000 2,000 0 2009 2010 2011 2012 2013 2014 2015 2016 2017 2018 2019 2020 Vessels constructed from 2009 to 2011 (greater than 5,000m3) Existing vessels (less than 1,500m3 or greater than 5,000m3) Existing vessels (between 1,500m3 and 5,000m3) Newly constructed vessels (greater than 5,000m3) Newly constructed vessels (less than 5,000m3) 2159 In the absence of IMO progress, the EU will be proposing a regional mechanism to reduce CO2 emissions from shipping. It remains unclear what the EU will eventually decide, although likely implementation of a3n0y3E4U plans is expected by 2017–2018 at the earliest, barring an IMO agreement. A last possible development in the international arena with regard to CO2 reductions is that shipping may be targeted to pay $5–6 billion per year into the UNFCCC-agreed Green Climate Fund. This fund is expected to disburse $100 billion per year to developing countries for climate change adaptation and mitigation purposes, starting in 2020. If agreed, it is likely that this will provide an impetus for the establishment of an IMO MBM that will generate the amount needed from shipping. However, as this will, most likely, necessitate a larger climate agreement at the UNFCCC by 2015, it is not presumed to have a significant impact on the industry until sometime after 2020. Figure 16: Estimated number of vessels required to install ballast water treatment systems. Source: IMO MEPC 61/2/17. Number of ships shipping 2020: 19 •••• Technology and energy efficiency Shipping will have to adopt new technologies over the next decade in order to be able to adapt to upcoming regulations and market pressure. The menu of available technologies is long – and filled with difficult decisions to make. Forthcoming regulations will force ship owners to address technologies that can impact SOx emissions, NOx emissions, ballast water cleaning and energy efficiency. The industry is developing solutions at a fast pace within all these areas. The development of new technologies is seen as impacting the whole value chain, from ship owners to suppliers and start-up companies. For each regulation, a ship owner will have multiple feasible technologies to choose among. Making the right choice will require knowledge of the effects, side-effects and operational implications. lise different processes. Technologies are emerging from different areas of the industry: land-based water treatment modified for shipping, new technology developed by shipping suppliers and the occasional start-up with a good idea. All systems, however, have relatively limited operational experience and come with an inherent technological uncertainty. Ballast water treatment technologies Many ballast water treatment systems are now available on the market and, with ratification of the IMO Convention awaiting, ship owners have an excellent opportunity to evaluate how these systems perform before the Convention enters into force. The careful selection of a treatment system is furthermore important in order to ensure that the system meets the ship-specific requirements, such as ballast water capacity, power limitations and the integration of control systems. Another important advantage is the opportunity to gain insight into the manufacturer’s commercial reliability, support network and quality of supply capabilities. to terminate the living organisms and for disinfection purposes, and can include the use of ozone, seawater electrolysis or electrodialysis, additives or a catalyst. These processes may not be efficient for larger organisms. There are numerous suppliers in the market providing approved systems that uti- Larger ship owners and smaller start-ups are likely to be at the forefront of the further development of ballast water treatment systems. However, the uncertainties are abundant, not least when it comes to which technologies to select and how the local regulatory landscape is likely to develop. The technology used will depend on the vessel type, trading pattern, vessel size and design. However, all ballast water management technologies involve a filtration process which separates particles from the water flow using membranes and hydro cyclones. The filtration measures are usually easy to install and maintain, and are often necessary to save sensitive components down the line. However, the process does create pressure drops and a reduction in flow rate. The filtration process is followed by mechanical/physical processes or active substances. The mechanical/physical processes can include cavitations, UV lighting, heat, vacuum, oxygen stripping or acoustic treatment. The physical process is often the main mechanism of the ballast water treatment system and may have a large power demand. Active/ chemical substances are added to the seawater Figure 17: A typical scrubber design; the physical dimensions can be seen in comparison with the main engine. Beyond the financial aspects, the requirement for space is a challenge. Source: Couple Systems GmbH. 20: shipping 2020 For each regulation, a ship owner will have multiple feasible technologies to choose among. Making the right choice will require knowledge of the effects, side-effects and operational implications. g NOx/kwh 18 16 14 12 10 8 6 4 2 0 0 Tier I – Year build of engine: 2000 Tier II – Year build of engine: 2011 Tier III (NOx Emission Control Areas) – Year build of engine: 2016 200 400 600 800 100 1200 1400 1600 1800 2000 Figure 18: NOx Tier I-II-III requirements. Source: IMO, MARPOL Annex VI. 2200 RPM SOx reduction technologies The revised Annex VI to MARPOL 2008 regulates the SOx emissions from ships, mainly by setting a limit for the sulphur content of marine fuel oils. Within specified ECAs, the sulphur limit will be even stricter than MARPOL Annex VI. removing nearly all sulphur from the exhaust, a scrubber also removes a large part of soot and particulate matter. However, the system takes up space, is a significant investment cost and requires additional energy to run. The technology has a rather limited track record aboard ships. Low sulphur fuel options will realistically be either expensive distillates or LNG, the latter in practical terms being an option mostly for newbuildings. For newbuildings from 2016 onwards and operating in an ECA, the NOx requirements add another layer of complexity due to possible technical incompatibility between SOx and NOx solutions. NOx reduction technologies NOx emissions are regulated through the revised MARPOL Annex VI 2008, which puts a limit on the specific emission from marine engines as a function of the revolutions per minute (rpm). The regulation applies only to newbuildings and is divided into three tiers based on the date of construction and on the operational area. Vessels with keel-laying dates after January 1, 2011 need to comply with Tier II requirements; these can easily be met by engine tuning by manufacturers. After January 1, 2016, newbuildings intended for operation in ECAs will have to meet Tier III requirements, which will require more drastic action. Feasible solutions include: ■■ Exhaust Gas Recirculation (EGR) ■■ Selective Catalytic Reactors (SCR) ■■ Water injection – Humid Air Motors (HAM)/Water in Fuel (WIF) ■■ LNG Based on a review of existing marine engine technology and expected technology developments, ship owners currently mainly have two choices if they wish to continue sailing in ECAs after 2015: install an exhaust gas scrubber or switch to low sulphur fuel including LNG. An exhaust gas scrubber can be installed to remove sulphur from the engine exhaust gas using seawater or freshwater and/or chemicals which are pumped through the scrubber. Dry scrubbers are also available, where hot exhaust gas is fed through a packed-bed absorber filled with lime in the form of granulate pellets which reacts with the SOx and produces gypsum, a soft sulphate mineral. The scrubber allows the ship to use cheaper, readily available high sulphur fuel. Besides Scrubbers are generally bulky and require alterations on board, such as additional tanks, pipes, pumps and a washwater treatment system. The sulphur is released overboard with the discharge washwater, and in open waters this is generally appreciated to be unproblematic from an environmental point of view. The sludge produced is categorised as special waste, to be disposed of at dedicated shore facilities. Scrubbers increase the power consumption by some percent, thereby increasing the total CO2 emissions. Scrubbers can be retrofitted to ensure ECA compliance for existing ships, although there is still some uncertainty about the consequences of scaling up such installations for large diesel engines. Exhaust gas recirculation (EGR), which is based on feeding exhaust gas into the combustion process, is regarded as a quite promising method, and engine makers currently have a strong focus on developing their EGR systems to ensure compliance with the strict Tier III requirements. The basic concept of the technology is that the higher heat capacity and lower oxygen content of the recirculated exhaust gas lower the peak combustion temperature significantly, which suppresses the formation of thermal NOx. EGR has few operational references, but the technology is expected to be commercially available as an alternative in the near future. Selective Catalytic Reactors (SCR) have already been installed on a number of ships and have proven to achieve significant emis- shipping 2020: 21 sion reductions. Certain issues have to be resolved, however, such as minimum exhaust gas temperature requirements to achieve the optimum effect and continuous operation of the catalyst. In addition, sulphur in the fuel has a tendency to pollute the catalyst material, so the SCR should ideally be installed after a SOx-reducing step, unless fuel with a low sulphur content is used. SCR is a proven technology and has been used in land-based power plants since the late 1970s, while the first marine application was introduced in the late 1980s. That said, the marine SCR system still needs to be matured, for daily and continuous marine operation using different fuel types and for compatibility with all engine types. Typical reduction levels of NOx are in the range of 50-95%, depending on the amount of urea (reducing agent) used, given that there is sufficiently high exhaust gas temperature to drive the process. Achieving sufficient exhaust gas temperature is a challenge for the operation of SCR systems at low engine loads and for two-stroke engines. Water injection (HAM/WIF) is an approach in which water is added through saturation of the scavenge air, as direct water injection or as emulsified water in the fuel. This is an effective way of reducing NOx emissions, although there is some concern that it may affect the engine’s thermal efficiency and cause fuel consumption to increase. The amount of NOx removed will depend on the amount of water injected: while emission reductions of up to 50% have been observed, emissions have typically been reduced by less than 30 percent. LNG as fuel LNG is currently installed as fuel on 30 vessels as of July, 2012, and there is a newbuilding order of approximately the same number of vessels. The technical challenges related to LNG as fuel have mainly centred on a few issues including: LNG handling and bunkering; and containment systems on board. Due to the very low temperature at which LNG must be transported, specialized alloys have been installed together with “traditional” tanks, pipes and machinery systems. Technical solutions continue to be researched and developed to permit further use of LNG as a marine fuel. Vessels currently built are covered by the IMO interim guidelines for LNG as ship fuel (MSC285(86)) and related class rules. This guideline gives the flag administrations the possibility to issue the necessary SOLAS certificates. This guideline will be replaced by the more general IGF-Code under development by the IMO with a target completion date in 2014. Technical challenges notwithstanding, the environmental benefits are significant. Use of LNG as fuel will reduce the NOx emissions by approximately 90% on a lean burn gas fuelled engine, and the SOx and particulate matters emissions are eliminated. The CO2 emissions are about 20% lower because of the lower carbon content of LNG. However, the release of unburned methane from engines (methane slip) is a challenge, especially for 4-stroke dual fuel engines, as the greenhouse gas effect of methane is between 20 and 25 times higher than for CO2. Regardless of a price premium of 15-20% compared to conventional engines, LNG fuel will become more relevant in the coming years for reasons related to both economies of scale and estimated lower fuel consumption. Energy efficiency and CO2 reduction technologies The first formal CO2 regulations were adopted by the IMO in 2011. As discussed in the previous chapter, these comprise the EEDI and SEEMP, both of which will enter into force on January 1, 2013. By setting increasingly stringent energy efficiency requirements for new ships, the EEDI is intended to stimulate the development of more energy efficient ship designs, thereby indirectly leading to reduced operational CO2/GHG emissions. The SEEMP, on the other hand, is designed to directly stimulate more energy efficient operational practices. Energy efficiency measures are different from other emission abatement technologies as they fulfil two purposes: they reduce fuel consumption and not emission directly, and they are (potentially) cost effective. The expected higher energy prices and corresponding fuel prices will increase the focus on development of more energy-efficient systems for ships. Ships built today may in the future compete with more efficient ships. The creation of various voluntary rating schemes for environmental performance, including CO2 performance, is providing tools that allow charterers and cargo owners to use only ships that satisfy their new and stringent requirements. Figure 19: Location of LNG fuel tanks on the Oshima Eco-ship. Source: DNV. Further, several technological measures will be increasingly used in the coming years. The options can be categorised into four groups, as shown below, although there are many different measures available for implementation in each category. ■■ Reduction in ship resistance ■■ Increase in propulsive efficiency ■■ Increase in power production efficiency ■■ Reduction in auxiliary consumption 22: shipping 2020 Reduction in ship resistance seems to be an area in which interest is only increasing in importance as the shipping industry prepares to address more fuel efficient solutions. The most common method of incorporating this concern is to optimize the hull design using more advanced computer tools. For some ship types, there may also be a potential for applying technology that impacts on hull resistance. This includes air cavity systems, which will impact on frictional resistance, and the use of lighter materials to reduce the lightweight of the ship. In addition, speed reductions will significantly reduce relative resistance and consumption per tonne-mile. Increasing propulsive efficiency has recently gained a great deal of attention due to the emergence of devices that may impact on this. Propulsive efficiency devices have the highest potential for effectiveness on ships with poor propeller working conditions and highly loaded propellers, but may be applied to all ship types. Increasing the propulsive efficiency has a direct impact on the total consumption and, as such, is an effective measure to reduce consumption across the complete operational profile. Power production on board a ship is based on two- and four-stroke engines. These engines have come close to their maximum potential in terms of efficiency. Systems that may increase the vessel’s total increase in power production efficiency have therefore attracted special notice. Among these, we find waste heat recovery, shaft generators and hybrid power systems. All of these will increase the complexity of the system, but may provide good efficiency benefits. Reductions in auxiliary power consumption can be achieved through improved power management, including efficiency measures such as waste heat recovery and improved transmission systems. Wind and solar power are technologies that also may offer fuel-free power production on ships. Both of these have received quite a bit of interest in the market, although they have only been implemented to a limited extent. A challenge with such systems is the increased complexity that they offer in design and operation. On the other hand, these are among the few that produce no emissions while in operation. Technology impact The technical measures evaluated in this report will enable ships to comply with future regulations in different ways. Figure 20 shows the motivating regulation for a technology and the expected maximum impact that the technology may have on compliance. As can be seen, not all technologies are able to completely fulfil their motivating regulations, and they may therefore have to be combined with other technologies in order to do so. For example, there are a good handful of technology options available to meet future CO2 regulations. TECHNICAL MEASURE Low sulphur heavy fuel oil SOx scrubber Distillate fuel Pure LNG engine Dual-fuel engine Exhaust gas recirculation Selective catalytic reduction Propulsion efficiency devices Waste heat recovery Shaft generators Hull shape optimisation Contra-rotating propulsion Air cushion Wind power Smaller engine/de-rating (speed reduction) System efficiency improvement Hybrid propulsion system Ballast Water Treatment System Water injection Water in fuel Low NOx tuning Lightweight constructions Reduction of seawater ballast capacity POSSIBLE FOR RETROFIT SECA 1%S SECA 0.1%S Motivating regulation GLOBAL 0.5%S NOX TIER III EEDI ENERGY BALLAST EFFICIENCY WATER 20 % 20 % 2 % 4 % 0-1% 5 % 4 % 6 % 2 % 10 % Considered, but not selected due to limited impact/effect Figure 20: Maritime technologies and relevant regulations. Source: DNV. shipping 2020: 23 •••• Fuel trends The shipping industry has become one of the major users of fossil fuels, and an increasing focus is being placed on the usage of maritime fuel due to increased scrutiny of the maritime industry’s emission levels. Fuel cost is the largest cost element for virtually every shipping company today. The regulatory shift towards low sulphur fuel is one of the developments in the industry that will have the largest impact in terms of shipping costs and operations, and may have a strong impact on the ‘uptake’ of new technologies. The viability of many emission reduction technologies depends heavily on various fuel prices and their relative differences. This factor, added to the overall importance of fuel prices for the profitability of the maritime industry, makes monitoring of the fuel markets and keeping track of their developments of significant importance. Maritime fuel – half of total voyage costs Developments in the maritime fuel industry have become crucial for operators and cargo owners as fuel consumption accounts for a large share of the total voyage costs and can constitute a significant portion of the total transportation costs. For the shipping industry, it is not surprising then that fuel costs, and consequently oil prices, are among the main drivers of the implementation of new technology. Shipping will be forced to reduce emissions through regulations. In the Sulphur Emission Control Areas (SECAs), which are North America, the Baltic Sea, the North Sea and the English Channel, the required sulphur content of fuel will be reduced from 1.5% to 1% beginning July 1, 2010, and to 0.1% beginning January 1, 2015. Globally, the highest permitted sulphur content of fuel will fall from 4.5% to 3.5% beginning January 1, 2012, and to 0.5% beginning January 1, 2020. Fuel grades currently in use on vessels fitted with sulphur scrubbers will still be permitted. Fuel type selection Increased environmental focus in today’s market and the simultaneous need for the shipping industry to become more accountable for its environmental footprint are influencing the decisions that shipping has to make in terms of fuel type selection. The growing scarcity and high price of oil will favour the use of renewable fuels. The IMO’s aim is to reduce emissions to air from ships, and ship owners must comply in one way or the other. The section on envi- ronmental regulations explains these principles in more detail. In this chapter, we have used energy and fuel price projections produced by the OECD, the International Energy Agency (IEA) and the US Energy Information Administration (EIA) which have been further developed by DNV Research & Innovation in the report entitled, “Maritime Fuel Price and Uptake Projections to 2035”. It should be noted that large price differentials in bunker fuels, and even more so with LNG, can be observed among different countries’ fuel markets. Heavy Fuel Oil To a very great extent, the variation in fuel oil prices is correlated to the movement of oil prices. If we compare the projections in the figure below with current prices, we see that the forecasted price is consistent with current levels that have been sustained since late 2009. However, we observe that the price ranges between $400 per tonne and $1,550 per tonne ($10.6 to 41.4 per MMBtu). Prices have been as low as $170 per tonne ($4.5/ MMBtu) as recently as March 2009, with an average that month of $350 per tonne ($9.35/MMBtu). Although it appears that, in the short term, prices are above even the high trend forecasts, it should be noted that these US$/tonne US$/tonne 1800 1600 1400 1200 1000 800 600 400 200 0 2010 2015 2020 2025 2030 2035 Low HFO Reference HFO High HFO Figure 21: HFO price projections 2010-2035 (real terms). Source: OECD, IEA, EIA Distillates. 24: shipping 2020 2500 2000 1500 1000 500 0 2010 2015 2020 2025 2030 2035 Low MGO Reference MGO High MGO Figure 22: MGO price projections 2010-2035 (real terms). Source: OECD, IEA, EIA. Following the new regulations, low sulphur fuel oil will be in great demand, which in turn will lead to a higher demand for alternative fuels. indicate trends and should not be taken as precise predictors of the price during a specific month or year. Marine Gas Oil (MGO) with 0.1% sulphur or less is readily available and shares more or less the same properties as the diesel fuel used for high-speed diesel engines. MGO results in low sulphur emissions, meeting the MARPOL Annex VI demands. MGO does not require any extra volume for storage tanks, and adjusting the engine to MGO requires in most cases only small investment costs. However, MGO fuel prices are higher than those of other heavy fuel oils due to its production process. In the projections used, MGO prices will range between approximately $500 per tonne ($12/MMBtu) and $1,500 per tonne ($37/ MMBtu) in 2015, and over $2,000 per tonne by 2035. The reference case for MGO shows an increase in real terms in the price from $1,100 per tonne in 2015 to approximately $1,200 per tonne ($29.5/MMBtu) by 2030. and availability of gas in LNG form in general. LNG availability differs from country to country and although the number of ships using LNG has been increasing, LNG engines are not yet commonly used on commercial vessels. LNG has to be stored in cryogenic tanks which require much more space than traditional fuel oil tanks. This may reduce the cargo capacity, depending on the type of vessel and the potential to have an adequate and safe location for the LNG tanks on board. LNG is assumed to be available at a competitive cost, but the future price level is highly uncertain. Like gas prices, LNG prices vary greatly from country to country. Based on the EIA and IEA projections the expected range in the price of LNG, which is likely to rise from the range of $300-800 per tonne ($7 to 17/MMBtu) in 2010 to the range of 400-1200 USD per tonne ($9 to 26/MMBtu) in 2035. The LNG price for marine use is likely to be on the high end of future price projections. to the profitability of the maritime industry, makes monitoring the fuel markets and keeping track of their developments of vital importance. Analysis of the oil and gas trends shows that gas and oil prices are expected to decouple and that shale gas is a game changer likely to increase spot gas availability in Europe. Following the new regulations, low sulphur fuel oil will be in great demand, which in turn will lead to a higher demand for alternative fuels. Liquefied natural gas In the past few years, LNG has become a more popular fuel for shipping. This development has much to do with the development of a distribution network and of the transport Looking ahead The viability of many emission reduction technologies depends heavily on various fuel prices and their relative differences. This fact, added to the overall importance of fuel prices US$/tonne 1400 1200 1000 800 600 400 200 0 2010 2015 2020 2025 2030 2035 Low LNG Reference LNG High LNG Figure 23: LNG price projections 2010-2035 (real terms). Source: OECD, IEA, EIA. shipping 2020: 25 26: shipping 2020 Chapter 2: Future scenarios In this chapter, we present four distinct scenarios concentrating on main global trends and drivers that affect the development of our industry. The trends and drivers are defined around two main axes: economic growth, and regulatory and stakeholder pressure. The trends and drivers themselves were described in the previous chapter, and each of these is, in our scenarios, weighted differently – giving us four distinct versions of what the shipping industry could look like in 2020. We have picked the year 2020 – distant enough to have plenty of uncertainty embedded in it, but close enough to have available predictions based on the current situation. Ultimately, we want to see how the various scenarios drive technology developments and associated investment levels and strategies in the maritime industry. © Getty Images INDEX •••• Why scenarios? 28 •••• Scenario A: “Full steam ahead” 30 •••• Scenario B: “Knowing the ropes” 32 •••• Scenario C: “Sink or swim” 34 •••• Scenario D: “In the doldrums” 36 shipping 2020: 27 Why scenarios? The scenarios describe likely outcomes on technology developments and associated investment levels and strategies in the maritime industry. A scenario is not a prediction of the future as such but rather a story of what the future might look like. With the scenario approach, we aim at spanning likely developments, at the same time as we want the scenarios to be sufficiently different to explore the effects of global trends and drivers in the shipping industry. respect to global growth. Regardless, the effects on shipping will be strong, as reflected in trade patterns, the required composition of fleets with respect to type and size of vessels, investment in newbuildings and scrapping rates. The trends constitute the platform for building our scenarios. The trends are based on forecasting and predictions, again rooted in historical data and extensive modelling, and use a number of reputable sources as well as trend analyses developed by DNV. The scenarios in turn tell stories that combine different trends. Four trends, each with a number of underlying drivers, are used when building the scenarios in this report: world growth and demand for seaborne trade; regulatory devel- opment as we move towards 2020 and beyond; technology developments; and finally, fuel developments. Looking at the current state of the shipping industry across segments and actors – owners, yards, manufacturers, banks, brokers and so forth, the development of these four trends will strongly influence, if not determine, the state of the industry in 2020. There is a naturally strong link between economic growth and demand for sea transport. We are currently in very uncertain times with In addition, the shipping industry is familiar with being regulated, and the world’s regulatory bodies have more in store in this respect. A number of new regulations, especially focusing on the environment and emissions from ships, are being shaped and may come into force over the next decade or so. These may directly affect the shipping business through increased costs and not least requirements regarding technology. The regulatory trend and the direction it takes will be a driver for innovation and technology development. LOW SCENARIO A: FULL STEAM AHEAD ECONOMIC GROWTH HIGH SCENARIO B: KNOWING THE ROPES High economic growth High fuel prices Little regulatory or stakeholder pressure on the environment High economic growth Cost of CO2 emissions up and on the rise in 2020 LNG prices decoupling from oil price and significantly lower Low economic growth LNG price decoupling from oil prices Little regulatory or stakeholder pressure on the environment SCENARIO D: IN THE DOLDRUMS Low economic growth Limited implementation of MBM results in a medium price on CO2 emissions Low fuel prices in general, but high demand keeps the MGO price up LOW SCENARIO C: SINK OR SWIM Figure 24: Four future scenarios modelled on economic growth and regulatory and stakeholder pressure. Source: DNV. 28: shipping 2020 HIGH REGULATORY AND STAKEHOLDER PRESSURE © Getty Images Where is technology going, and what options are available? Are available options proven for safe and reliable operation? What are the investment costs and not least operating expenses? These are the questions the industry must grapple with and find good answers to in the short to medium term. Fuel costs represent a large share of the operating costs – up to 80% on larger container vessels. Regarding fuel price projections, one common view is that high oil prices are here to stay. Whether LNG can be a game changer as a shipping fuel will depend on price and on availability. Each of our four scenarios, representing combinations of the trends and drivers as outlined, defines a complete set of input to our simulation model. The results generated from the model complete our narrative with a particular focus on technology ‘uptake’ and the industry options. The four scenarios illustrated in Figure 24 are described in detail in the following pages, while the chapter closes with a table summarizing the scenarios in more quantitative detail. shipping 2020: 29 •••• Scenario A: “Full steam ahead” High economic growth, low regulatory and stakeholder pressure. In this scenario, the world is in a strong growth period in 2020, and shipping industry experience resembles the boom periods of the past. Yesterday’s shipping technology prevails. Global environmental regulations are in a stalemate after a decade of negotiations and wrangling. There is less focus on caring for future generations; short-term problem solving and “living for the moment” are what matter. The world economy is in good shape, and the growth rate continues to be high, at 4% annually in the Western world including the Eurozone, and at 8% annually in China and India in 2020, while these figures may increase further in the future. World demand for natural resources is escalating. This is causing an increase in exploration and production which has strong positive effects for seaborne trade, including the transport of manufactured goods. In this scenario, trade growth doubles compared to world growth. This is consistent with historical periods of high growth. Inter-Asia trade growth is very strong, with annual volumes shipped tripled by 2020 compared to 2012. This has a strong impact on, for example, the midsize container segment, the bulk segment and associated port facilities. The expanded Panama Canal has positive effects on trade patterns such as US West Coast-East Coast container trade. Overall, we estimate the size (dwt) of the 2020 world fleet to be 50–60% greater than the 2012 level. World growth is dependent on readily available fossil fuel, with coal still dominant and relatively cheap compared to oil. The high price of energy remains a threat to growth but is also a consequence of high demand. The prices of the three alternative types of shipping fuels – HFO, MGO and LNG – follow the oil price, and as such all three remain at a high and increasing level in 2020 and beyond. MGO remains the most expensive of the three, at approximately 30% above the HFO price, with LNG at approximately 10% above the HFO price (including bunkering cost). SCENARIO A: FULL STEAM AHEAD ECONOMIC GROWTH HIGH High economic growth High fuel prices Little regulatory or stakeholder pressure on the environment REGULATORY AND STAKEHOLDER PRESSURE LOW HIGH LOW There is low regulatory and stakeholder pressure. There is low regulatory and stakeholder pressure, and we see a negative trend with regard to the further development of global regulation. There has been a tendency to see more regional and local regulatory initiatives, but some of these are also losing momentum. One example of this is that the EU’s plan for implementing ECA-like requirements in all EU waters is put on hold and the discussions seem to lose ground and fade out. The 0.5% sulphur global cap planned for 2020 is postponed until 2025. The proposed plan of having the shipping industry contribute to the United Nations Framework Convention on Climate Change (UNFCCC)-agreed Green Climate Fund is also shelved. The Ballast Water Management Convention (BWMC) has not entered into force.The US decides not to progress with its own ballast water cleaning standards, planned to be stricter than IMO standards, but enforces the IMO requirements in their own waters. There has been no success in implementing MBMs, mainly due to major disagreements on the applicability of these mechanisms. The EU has also been unsuccessful in implementing regional mechanisms to reduce CO2 emissions from shipping. In a world where there is low regulatory pressure, greenhouse gas emissions are “free” and there is robust growth in seaborne trade: shipping thrives in its traditional way – risk capital is available, there is strong fleet growth with standard designs and scrapping rates are low. Still, technology and innovation represent competitive advantages for owners, yards and manufacturers. In 2020, the leading players are driving developments that exceed the level of environmental regulations in place, branding themselves above the minimum standards. The level of R&D in shipping is twice as high as 2012 levels. 30: shipping 2020 © Getty Images shipping 2020: 31 •••• Scenario B: “Knowing the ropes” High economic growth and high regulatory and stakeholder pressure. In this scenario, the world is in a strong growth period and the shipping industry is experiencing a time similar to the boom periods of the past. There have been breakthroughs with regard to binding international agreements on SOx, NOx and CO2 emissions and ballast water regulations. This causes global environmental regulations to have a strong influence on the shipping agenda. Corporate Responsibility is a boardroom topic in shipping companies. With growth rates of 4% in the Western world and 8% in China and India, the world economy is strong. The exploration for and production of natural resources is increasing due to strong economic growth. This has a substantial effect on seaborne trade, especially the bulk and container markets. Trade growth doubles compared to world growth. In this scenario, Inter-Asia trade is expected to triple by 2020 compared to the 2012 level. This has a strong impact on the midsize container segment, bulk segment and associated port facilities. ECONOMIC GROWTH HIGH SCENARIO B: KNOWING THE ROPES High economic growth Cost of CO2 emissions up and on the rise in 2020 LNG prices decoupling from oil price and significantly lower HIGH LOW REGULATORY AND LOW STAKEHOLDER PRESSURE Overall, we estimate the size (dwt) of the 2020 shipping thrives through a high degree of innovation world fleet to increase by 50-60% compared to the 2012 level. and technology development. The prices of HFO and MGO follow the oil price and remain at a high and increasing level by 2020 and beyond. MGO is 150% more expensive than HFO, while the price of LNG is decoupled from the oil price due to rising production and gas price developments in general. LNG is an attractive ship fuel from a price viewpoint with the LNG price per tonne at 30% of the HFO price. LNG bunkering networks (terminal and barges) are being developed across main bunker locations and in locations with strong trade growth. Innovation and technology developments relating to LNG as a fuel are strong. We have a legally binding agreement on global cuts in CO2 emissions that includes all countries, including the US and China. The EEDI scheme has been further developed beyond its 2013 introduction and is mandatory for existing ships. We expect to observe a forced phase-out of energy-inefficient ships, similar to what we saw for single hull crude oil tankers. There are major commercial implica- tions for shipping companies and yards and these are strong drivers for innovation and technology development in the shipping industry. In 2020, ECAs cover all coastal areas worldwide. There are no ‘sanctuaries’ to be found for ships emitting SOx, NOx and PM. The transition to low sulphur fuels, in particular LNG, is shipping’s strongest trend. The BWMC has been ratified to a level covering 80% of merchant shipping, and is a strong driver for technology uptake in this area. There has also been some success in implementing MBMs, and the global cost of CO2 emissions is effectively in the range of $50 to $100/tonne. In a world where there is high regulatory and stakeholder pressure combined with strong growth in seaborne trade, shipping thrives through a high degree of innovation and technology development. We see an increased focus on environmental performance by charterers, forcing ship owners to implement environmentally friendly technology. R&D funding is tripled compared to 2012 levels. Retrofitting in order to meet regulations and support environmentally friendly shipping is a booming industry. Investment in technology and innovation in this area is, for commercial reasons alone, seen as a way of gaining a competitive advantage. Integrating sustainability and environmental issues into company strategy is seen as a differentiator; opposing initiatives have a huge downside and include the risk of tarnished reputation. 32: shipping 2020 ©Getty Images shipping 2020: 33 •••• Scenario C: “Sink or swim” Low economic growth and high regulatory and stakeholder pressure. In this scenario, the world is experiencing a turbulent economic situation and the future does not look promising. The crisis in the Eurozone related to unsustainable sovereign debt levels has deepened and growth in the Western world is very modest, at approximately 2% in 2020. China and India have similar growth levels, at approximately 5% in 2020. For China, in particular, this represents a somewhat ‘hard landing.’ In both China and India, percentage growth in the population moving into the middle class has declined, and anticipated growth in Inter-Asian trade is quite modest. Still, the BRICKTIM countries constitute 40% of the world population and have outgrown the largest economies of the past. Yard capacity is at an all-time high, order books are empty and the yards are suffering as a result of low newbuilding activity. They are more than willing to develop innovative solutions. However, there are few buyers, apart from some risk-willing first movers, due to limited capital availability. As a consequence, the yards’ main focus is on retrofitting. Overall, we estimate the size (dwt) of the 2020 world fleet to be 25–30% higher than the 2012 level. HIGH LOW Low economic growth Limited implementation of MBM gives a medium price on CO2 emissions Low fuel prices in general, but high demand keeps the MGO price up LOW SCENARIO C: SINK OR SWIM ECONOMIC GROWTH HIGH REGULATORY AND STAKEHOLDER PRESSURE Poor economic conditions limit the funding of new technology. At the same time, environmental awareness is rising The prices of HFO, MGO and LNG follow the oil price, and as such all three remain at a high and increasing level in 2020 and beyond. MGO remains the most expensive of the three, at approximately twice the HFO price, with LNG at approximately 10% above the HFO price (including bunkering cost). Poor economic conditions limit the funding of new technology. At the same time, environmental awareness is rising and there is an increased focus on the problem of pollution from the maritime sector. We have experienced accelerating global temperatures due to greenhouse effects and severe consequences in terms of flooding in several parts of the world. Strong local and regional regulatory initiatives are supported. One of the few successful global initiatives is the BWMC, which was ratified in 2014. This initiative requires most ships in international trade to install ballast water cleaning systems by the end of 2019. The implication is that several thousand ships will need to have systems installed within a very short time span. The yards position themselves to get the work of installing the systems, but the costs concern owners. Furthermore, there has been limited success in implementing MBMs, and the global cost of CO2 emissions is effectively in the range of $30–50/tonne. A world where there is low GDP growth but high regulatory pressure is a bleak scenario for yards and owners alike. Low capital availability in the market puts limitations on the funding of new technology. Still, yards and manufacturers see opportunities coming from the high regulatory and stakeholder pressure, and some first movers will invest in innovation as a means to gain a competitive advantage. 34: shipping 2020 shipping 2020: 35 © DNV/ Nina E. Rangøy •••• Scenario D: “In the doldrums” Low economic growth and low regulatory and stakeholder pressure In this scenario, we predict stormy times worldwide, and the economic situation is fragile. Growth in the Western world is low, at approximately 2 percent. China and India have similar growth levels at 5% annually. The percentage growth of the Chinese middle class is lower than anticipated, and the estimated growth in Inter-Asia trade has not been as high as projected. The bulk and container markets suffer the most. Few new vessels are built and the scrapping rate is high. Times are tough for yards and manufacturers as demand is low. Many of them are on the verge of bankruptcy and competition is fierce. For most of the yards, the main focus is on retrofitting. REGULATORY AND LOW STAKEHOLDER PRESSURE Prices of HFO and MGO follow the oil price and stay at a high and increasing level in 2020 and beyond. MGO remains the most expensive, at approximately 50% above the HFO price, whereas LNG is decoupled from the oil price and trades at approximately 70% of the HFO level. Low economic growth LNG price decoupling from oil prices Little regulatory or stakeholder pressure on the environment HIGH HIGH SCENARIO D: IN THE DOLDRUMS LOW ECONOMIC GROWTH Overall, we estimate the size of the 2020 world fleet to be 25-30% larger than the 2012 level. With weak economic conditions and fewer market interventions, governments are choosing more protectionist tactics and policies that mainly focus on protecting their own national interests. This has led to less focus on global collaboration with regard to environmental regulations. There are few incentives to introduce new regulations and strengthen existing ones. Many schemes and initiatives are therefore being shelved. The only exception to this is the global cap on SOx emissions, set for 2020. The United States decides not to introduce its own ballast water cleaning standards. Instead, the US ballast water requirements become aligned with the less stringent IMO requirements. less focus on global collaboration with regard to environmental regulations In this scenario, ship owners, yards and manufacturers are experiencing tough times and the banks are also concerned. We do not see any prospects of change in the foreseeable future. There is limited capital available for technology, R&D and education due to the weak state of the industry and the low regulatory and stakeholder pressure. Consequently, most innovations remain on the drawing board. MBMs are losing momentum and the EU is unsuccessful in implementing regional mechanisms to reduce CO2 emissions from shipping. The plans for universal coverage of all vessels trading in Europe are not implemented. 36: shipping 2020 © Getty Images shipping 2020: 37 38: shipping 2020 Chapter 3: Technology investment Ships are long-lived assets. A ship can trade in the market for 20 to 30 years. In an uncertain future, it will have to be flexible enough to deal with upcoming requirements. From an historical perspective, technology uptake in shipping has been a slow process. In this chapter, we have taken a closer look at ship owners’ investment profiles. Our findings are based on the results of a survey conducted among ship owners, investigating which key parameters and prioritisations are important when investing in a new vessel. © Getty Images INDEX •••• rTegchunlaotolorgyytriennvedstment 04X0 •••• technology trends 0X •••• fuel trends 0X •••• world economy and demand for shipping 0X shipping 2020: 39 •••• Technology investment The decision on whether or not to invest in a new ship and what kind of technology it should contain is in the hands of the ship owner. The ship owners’ decisions are influenced by both commercial and regulatory factors. History shows that major technology updating in shipping is driven primarily by regulatory changes as a consequence of major accidents. Furthermore, in an increasingly competitive business, operating costs and fuel prices in particular are influencing the ship owner’s decisions. Technology uptake also depends on the degree of which a technology is implemented, as financial and information costs tend to decrease as technologies mature. Not surprisingly, one of the findings of our survey was that new regulations are major drivers in technology uptake, as these have to be met in order to be able to operate. Furthermore, cost-reducing measures and the flexibility of the vessel are important considerations. Historical uptake of new technologies History shows that innovation is a slow process in the shipping industry. The containerisation of shipping started in the 1950s, but it was not until global standards were fixed in the late 1960s that it gained traction. After that, it took 30 years to take over 90% of the general cargo market. Another example is the implementation of double hulls on tankers. This started with chemical carriers in the 1970s and 1980s. After major oil spills such as the Atlantic Empress/Aegean Caption collision in 1979 and the Exxon Valdez in 1989, the discussion about double hulls for all tankers started, but it was not until the US Oil Pollution Act in 1990 and global MARPOL regulations in 1992 that double hulls became mandatory. Again, it took almost 30 years for double hulls on all newbuildings to achieve a 100% market share. The uptake of new technology typically follows an S-shaped curve, with a slow initial acceptance followed by an accelerating pace, when the majority start implementing and before it eventually slows down, as the market becomes saturated. Regulations and increasing competitiveness are major drivers of technology implementation in the maritime industry. Environmental technologies are driven primarily by new regulations, the exception being energy efficiency measures which can increase the competiveness of a ship. In any case, these technologies are implemented slowly due to a range of barriers such as lack of capital, split incentives on fuel savings, yard and designer capacity, and uncertainty connected to new and unproven technology. In February 2010, DNV published “Pathways to low carbon shipping”, a report which was a study of the potential for CO2 emission reductions in the world fleet and the cost effectiveness of the technologies, looking forward to 2030. As can be seen in Figure 27, a large amount of the possible reduction in CO2 is shown to be cost-effective. This raises the question of why so few of these measures have been implemented when, meanwhile, the fuel price level has more than doubled within a few years. Part of the answer is either that many ship owners and operators actually have implemented the measures, or that the full reduction potential has not been achieved. In addition, the barriers to implementation are not only technical; financial and organisational obstacles hinder the uptake as well. In particular, many operational measures Market share (new build dwt) Market share (new build) CONTAINER VS DRY CARGO TONNAGE 100% 80% 60% 40% 20% 0% 1960 1970 1980 1990 Year 2000 2010 Figure 25: Container vs dry cargo tonnage market share. Source: IHS Fairplay. 40: shipping 2020 DOUBLE HULL DIFFUSION – TANKERS ABOVE 5000 DWT 100% 80% 60% 40% 20% 0% 1960 1970 1975 1980 1985 Year 1990 1995 2000 Figure 26: Double hull market share – tankers above 5,000 dwt. Source: IHS Fairplay. Regulations and increasing competitiveness are major drivers of technology implementation in the maritime industry. Cost per ton CO2 averted ($/tonne) 220 180 140 100 60 20 -20 -60 -100 0 Voyage execution Steam plant operational improvements Speed reduction (port efficiency) Engine monitoring Reduce auxilitary power Propulsion efficiency devices Trim/draft Frequency converters Propeller condition Contra-rotating propellers Weather routing Air cavity/lubrication Hull condition Kite Solar panel (not shown) Wind generator (not shown) Gas fuelled Electronic engine control Light system Fuel cells as aux engine Speed reduction (fleet increase) Fixed sails/wings Waste heat recovery Exhaust gas boilers on aux Cold ironing 100 200 300 400 500 600 700 800 CO2 reduction (million tonnes per year) Baseline 1,530 million tonnes per year Figure 27: Pathways to low carbon shipping. Abatement potential towards 2030. Source: DNV, February 2010. require an organisational change and continuous effort, rather than a simple installation of a technology. This Shipping 2020 study focuses on the technology options and does not include an analysis of operational measures, and thus organisational barriers are not addressed. The financial aspects of a technology investment, however, are analysed in more detail, together with more insight into different operational aspects. Ship owner survey Each ship owner will have an investment policy that depends on market conditions, willingness to take risk, area of operation and type of trade. One example of such a factor is time spent in an ECA. Figure 28 shows the share of time different ship types spend in an ECA, based on AIS data for the Baltic and North Sea in 2010. The time spent in an ECA may affect the choice of technology. For instance, it is less costly to invest in a SOx scrubber than to use LNG as fuel if the ship spends little time trading within an ECA. The required payback time is a crucial decision parameter for many ship owners. Some have a long-term perspective and may invest in technologies that take many years before they result in positive returns on investment, while others are more concerned about shortterm profit and the second-hand value of the ship. A technology which is profitable in the Small RoRo General Cargo Offshore - Other Small Container Large RoRo Offshore - PSV Small tanker Large Tanker Offshore - AHTS Small Bulk Medium tanker Large Bulk Large Container 0% 26-100% 1-26% 0% 20% 40% 60% 80% 100% Figure 28: Relationship between time spent in ECA and ship type. Source: AIS data 2010, DNV analysis. shipping 2020: 41 FUEL PAID BY SHIP OWNERS INDUSTRIAL PLAYERS Typical contracts and ship segments: • Contract of affreightment • Liner operations • Tanker and bulk carrier voyage charters • Roro/vehicle carriers Important investment factors: • Short- and long-term charter rates, fuel costs • 30% of the industry Typical contracts and ship segments: • Voyage charters in offshore • Time charters Important investment factors: • Short- and long-term charter rates, probability of obtaining charter • 60% of the industry • Very few players in this category Typical contracts and ship segments: • Bare boat charters Important investment factors: • Second-hand value of ship, long-term charter rates, probability of obtaining charter • 10% of the industry FUEL PAID BY CHARTERERS ASSET PLAYERS Figure 29: Typical characteristics of players in the shipping industry. Source: DNV, 2012. eyes of one owner may not be so for another. As part of this report, DNV conducted a survey during Spring, 2012, exploring ship owners’ attitudes and investment choice characteristics. The survey was distributed to approximately one hundred and eighty individuals, whereof twenty-four responses were received. Of these respondents, sixteen were positioned in senior management and the remaining eight were employed in a technical department. According to our survey, 75% of the respondents have a payback horizon of 0–5 years, while 25% have a horizon of only 0–2 years. In the current situation, with many new regulations in the pipeline and rising fuel prices, the second-hand value of a ship becomes a prominent factor which is again dependent on the ship’s fuel efficiency and transport effectiveness. A ship with low fuel consumption is more attractive in the marketplace and can expect to be more likely to obtain a charter in the future. However, historically, efficient ships have not been able to obtain a higher rate. This may change. PAYBACK TIME 17% 8% 25% 50% 0–2 years 3–5 years 6–10 years No particular requirements to payback time FUEL EXPENSES 9% 26% 35% 8% 22% The charterer pays 0% of the fuel expenses The charterer pays 51-75% of the fuel expenses The charterer pays 76-99% of the fuel expenses The charterer pays 100% of the fuel expenses I don’t know Figure 30: Shipowners’ expected payback time (investment horizon). Source: DNV, 2012. 42: shipping 2020 Figure 31: Fuel cost burden of ship owners and charterers. Source: DNV, 2012. © Getty Images shipping 2020: 43 Further, those companies having the strongest incentives for investing in new fuel saving technologies are the ones who pay their own fuel bills. According to our survey, the following describes an approximate picture of the distribution of the fuel expenses: For a ship owner, a decision to invest in additional technologies will usually fulfil at least one of the following criteria: ■■ Make the vessel compliant with a regulation or requirement ■■ Reduce the fuel cost paid by the ship owner ■■ Increase the expected charter rate ■■ Increase the probability of obtaining a charter contract ■■ Increase the second-hand resale value Results from the DNV survey indicate that regulations and cost efficiency are the most significant motivations for investing in new technology, while the costs of installation and operation and the maturity of the technology itself are the most important barriers to implementation. Figure 32 shows how typical trades and segments operate, dividing ship owners into asset players and industrial players. Assets players are most prominent in bare-boat chartering and do not operate ships on their own. These players are mainly concerned with winning long-term contracts and may invest in technology to increase the probability of obtaining a charter, but do not pay the fuel costs, and the profitability of a fuel effi- ciency measure is therefore limited. The industrial players are directly involved in the management and operation of the ship. In voyage charters, liner operations and contracts of affreightment, the ship owners are paying for the fuel and have a strong incentive to increase the energy efficiency. Owners that are operating in the spot market with time charters are less involved in the operation of the ships and focus on the technical management. Since they do not pay the fuel costs themselves, the motivation for fuel efficiency is mainly to be as attractive as possible for chartering. The results of the survey illustrate that the ship owner’s investment horizon varies from very short to long term among various industrial players. The respondents in our survey are mainly ship owners. Of these, 65% report that the charterer covers more than 50% of the fuel expenses. Furthermore, 35% of the respondents report that the charterer covers 100% of the fuel expenses. A ship owner that covers the fuel cost does not necessarily have a longer investment horizon. To get a clear overview of our respondents, we asked them whether they had tested or were familiar with different technologies. The feedback, presented in Figure 34, show a discrepancy between familiarity and testing. Low sulphur HFO, distillates and shaft generators show the highest scores when it comes to both testing and familiarity. The respondents seem to be quite familiar with ballast water treatment systems, but report low scores when it comes to testing this technology. The respondents are least familiar with wind and solar power and humid air motor and air cushion technologies. The technologies that score the lowest on testing are humid air motor, air cushion and exhaust gas recirculation systems. In the survey as shown in Figure 35, the respondents were also asked to rank how likely it was that specific technologies would be implemented in existing and new ships. The most likely was a ballast water treatment system, followed by low sulphur heavy fuel or distillate fuel for existing ships, all of which will be mandated by regulations. Regarding energy efficiency, hull optimisation and waste heat recovery received high scores, especially for newbuildings and propulsion efficiency devices, while auxiliary system improvements and, to some degree, waste heat recovery are more likely to be implemented on existing ships. When it comes to NOx reduction technologies, the responses do not give a clear indication of the preferred choice. However, exhaust gas recirculation, selective catalytic reduction and gas-fuelled engines do not achieve high scores. Comply with current/future rules & regulations Become more fuel efficient, increase competitive situation (more cost effective) Trade flexibility (ECA friendly vessels) Branding, innovation, first-mover perception 1 2 3 Low Score 4 5 High Figure 32: Motivation for implementing new technologies. Source: DNV, 2012. 44: shipping 2020 Cost of installation/purchase Cost of operation Technical maturity/reliability/experience Lack of competitive (market, financial) incentives Safety of crew and ship Complexity of operation (after installation) Complexity of installation and system integration Specific know-how among crew to operate/maintain Not compatible with current charter agreements Acceptance from Class towards new technologies 1 2 Low 3 4 Score 5 High Figure 33: Barriers to implementing new technologies. Source: DNV, 2012. Ballast Water Treatment System Low sulphur heavy fuel oil System efficiency improvement (Aux) Hull shape optimization Waste heat recovery Propulsion efficiency devices Distillate fuel EGR system Low NOx tuning Shaft generators Smaller engine/de-rating (speed reduction) Reduction of seawater ballast capacity SCR system SOx scrubber Lightweight constructions Dual fuel engine Water emulsification Humid air motor/ direct water injection Hybrid propulsion system Counter rotating propulsion Pure gas engine Air cushion Wind & solar power 1 Low 2 3 4 Score 5 High Likely to be retrofitted Likely to be implemented on newbuildings Figure 34: Survey respondents’ likelihood of implementing specific technologies. Source: DNV, 2012. Low sulphur heavy fuel oil Distillate fuel Shaft generators Ballast Water Treatment System Waste heat recovery Propulsion efficiency devices Hull shape optimization SOx scrubber System efficiency improvement (Aux) Smaller engine/de-rating (speed reduction) Low NOx tuning Reduction of seawater ballast capacity EGR system Counter rotating propulsion Dual fuel engine SCR system Hybrid propulsion system Pure gas engine Water emulsification Lightweight constructions Air cushion Humid air motor/ direct water injection Wind & solar power 1 Low 2 3 4 Score 5 High Familiarity with technology Tested Figure 35: Survey respondents’ familiarity with specific technologies. Source: DNV, 2012. shipping 2020: 45 46: shipping 2020 Chapter 4: Methodology and assumptions DNV has developed a simulation model to determine which technologies are likely to be implemented in the period leading up to 2020. The model takes into account a broad range of variables, such as investment horizons, fuel burdens, operational patterns and risk appetite within the industry. The model does not try to optimize the best path ahead, but simulates how each ship owner individually will seek to comply with regulations and increase energy efficiency. This chapter outlines the methodology and assumptions made. © Getty Images INDEX •••• Methodology and assumptions 48 shipping 2020: 47 •••• Methodology and assumptions DNV has developed a stochastic simulation model to analyse technology uptake in four future scenarios. The scenarios also outline alternative outcomes on development given regulatory requirements and fuel price fluctuations. The assessment of technology uptake presented in this study is based on a stochastic simulation model that simulates technology investment decisions in the world fleet for the time period 2012–2020. The model is used to analyse a set of future scenarios representing alternative outcomes about development in regulatory requirements and fuel prices. Simulation model DNV has developed a simulation model to determine which technologies are likely to be implemented for the period leading up to 2020. We use a bottom-up approach and simulate newbuilding and retrofit technology decisions for a representative set of ships, using regulatory compliance and net present value (NPV) as the main decision criteria. In a simulation run, the model initially generates a sample of individual ships that is representative for the operating fleet at the end of 2011. Each ship is given specific technical characteristics and owner preferences drawn from statistical distributions representing the diversity of the world fleet and its owners. The model then steps through each year until 2020. In each year, newbuildings are added to the fleet and older ships are scrapped. For each ship, newbuilding and existing, the model simulates the decision to install one or more technologies. The resulting technology uptake from a simulation run is a result of these decisions. For each scenario, a large number of simulation runs are undertaken to see the effect on technology uptake of fuel prices and technology costs. Figure 36 shows the simulated fuel price paths for a given simulation run. Due to the diversity of the fleet and owner characteristics, some ships decide to invest in technology, while others do not. For each scenario A–D, the model generates a large number of simulation runs to adequately represent the uncertainty in fuel prices and technology cost. The technology uptake over time is therefore uncertain. An important feature of the model is that the outcome of an investment decision is time dependent, and may lead to technology lockin effects as well as positive feedback effects on subsequent technology uptake. This is due to the following: 1. Simulated fuel prices are uncertain and time dependent; 2. Technology decisions may limit available options for later decisions due to technology incompatibility; and 3. The cost of a technology is reduced from learning, as more and more ships adopt it. It is noted that learning will occur across fleet segments. This implies that the initial cost reduction from early adoption of a technology in one segment (e.g. due to regulations) may trigger a cascade of technology adoptions across the fleet. Investment decision criteria The technology investment decision is evaluated on an annual basis (at year start) for each ship being simulated. Based on fuel prices, technology costs and specific ship/ owner characteristics, the model selects the technology or technologies with the highest NPV that ensures compliance with the relevant SOx, NOx, ballast water and EEDI requirements. Only regulatory requirements that will enter into force the year the decision is made (the year of delivery in terms of newbuilding) are considered relevant. The model does not consider any uncertainty related to regulations outside the differences in assumptions in the scenarios. After compliance has been secured by selecting the most cost effective technologies, all remaining technologies with positive NPV are added. The NPV is calculated based on the ship specific discount rate and investment horizon, the simulated investment cost, and simulated fuel prices for the current year. When the model takes an investment decision, fuel prices are assumed constant over the investment horizon. The investment horizon is assumed to be similar to the required payback period. The discount rate and investment horizon for each ship is assumed constant for the simulated period, 2012–2020. The second hand value of the ship is not included in the NPV calculated for each technology. For each year and for each ship, the model will select the fuel type that gives the highest NPV and that meets the regulatory requirements for that year, given the range of possible fuels for that ship – e.g. a dual fuel engine will enable the ship to run on LNG and HFO. Fuel prices The model simulates uncertainty in prices for HFO, low sulphur heavy fuel oil (LSHFO), MGO and LNG. This is done by utilising a relative simple mean reversion stochastic model that simulates uncertainty around the fuel price trends defined for each scenario. Uncertainty in fuel oil prices is assumed to be linked to the crude oil price. There are two possibilities for simulating LNG price uncertainty in the model: 1. As fully linked to crude oil price; and 2. As a separate gas market with no oil link. Historical spot price data (1997–2012) for Brent crude and Henry Hub natural gas is used to parameterise the stochastic model. Ship and owner characteristics A ship in the model is defined by a range of quantitative parameters, such as energy consumptions per year, estimated time in ECA per year, share of fuel cost paid by owner, newbuilding year, energy efficiency, discount rate, and investment horizon, i.e., the ship owner’s preferences in terms of economic horizon and required return on investment. 48: shipping 2020 Based on fuel prices, technology costs and specific ship owner characteristics, the simulation model selects the technology or technologies with the highest NPV that ensures compliance with the relevant requirements. Simulate fuel prices on a monthly basis until 2020 Generate a representative sample of the current world fleet Simulate annual retrofit and fuel decisions for all existing ships until 2020 MGO HFO ECA 0.1% SOx LSHFO Improve sys. eff. Improve prop. eff. LSHFO 2012 2013 LSHFO 2014 ECA Tier III NOx ECA 0.1% SOx EEDI Phase 1 LNG LNG LNG Dual fuel engine 2015 2016 2017 LNG Global 0.5%SOx EEDI Phase 2 LNG LNG LNG 2018 2019 2020 Add newbuildings to the fleet each year Simulate annual retrofit and fuel decisions for all newbuildings until 2020 Remove scrapped ship from simulated fleet each year Figure 36: Example of simulation run. The figure aims to illustrate the simulated fuel price paths for a given simulation run. Due to the diversity of the fleet and owner characteristics, some ships decide to invest in technology, while others do not. For each scenario A–D, the model generates a large number of simulation runs to adequately represent the uncertainty in fuel prices and technology cost. Source: DNV. The parameter value is set by sampling from a statistical distribution that represents the heterogeneity (diversity) in the fleet segment for that particular parameter, e.g. time in ECA or discount rate. With a sufficiently large number of ships in the model, the variation across the simulated fleet is representative for the variations observed in the actual fleet. Technology investment cost and compatibility In total, 23 technologies are assessed in quantitative terms. Some technologies have been assessed but dismissed from the modelling and simulation work as they are considered to be less cost effective or too immature at the moment. We have also been forced to make some simplifications regarding the technologies, as different stakeholders claim different effects and operational characteristics. The technologies have been quantified in terms of: 1. Costs/CAPEX and assumed energy and emission reduction effect 2. Regulatory compliance 3. Compatibility and overlap between technologies A base estimate for technology investment cost (CAPEX) is specified for each fleet seg- ment, with an uncertainty factor that is given per technology. CAPEX uncertainty varies from +/-5% to +/-30 percent. The relative initial price difference between technologies is sampled for each simulation run. However, due to learning, investment cost is reduced over time as more and more ships adopt a technology. Hence, the relative price difference between technologies may change over time within a simulation run. Learning is assumed to occur across segments, meaning that uptake in one segment will lead to reduced price in all segments. The learning factor is estimated at 10–20%; meaning a 10–20% price reduction is projected each time the number of technology adoptions is shipping 2020: 49 doubled. The estimated cost of each technology also did not include losses related to time off hire. The different technologies assessed in this project are expected to be used in order to meet future regulations, and their compatibility is a critical cornerstone of the modelling work. We have created compatibility matrices for all the relevant technologies. There are several types of compatibility and incompatibility of note: ■■ Compatibility may be seen as a technical issue, where space, location and technical performance are important parameters (e.g. EGR works well with distillate fuel). ■■ Compatibility may be seen as overlapping technologies, where, for example, two technologies are needed in order to meet the regulations (e.g. to meet EEDI regulations, both hull optimisation and propulsion efficiency devices may be needed). ■■ Incompatibility may be present when it is impossible to implement two types of technologies at the same time (e.g. distillate fuel and LSHFO) for technical reasons. ■■ Incompatibility may also be present when one technology has a mitigating effect on another (e.g. distillate fuel and a SOx scrubber), or where the first technology is sufficient to secure compliance and a second technology is therefore redundant. The possible constraint on technology uptake resulting from lack of yard and/or maker capacity is not included in the analysis. Model implementation The model was implemented by DNV using the simulation software environment, ExtendSim (AT 8.0.2). ExtendSim is a powerful module-based simulation modelling and analysis environment. The model developed in this study utilised discrete event simulation and Monte Carlo sampling. Input and output data was managed by ExtendSim’s built-in database functionality. Simulation results were exported to MS Excel for further analysis and graphical presentation. Other assumptions and simplifications A wide range of assumptions has been used in the modelling work, the most important being the following: 1. The world fleet composition is based on the updated IMO GHG study and IHS Fairplay World Fleet Database. The fleet is divided into 13 segments and includes all cargo-carrying ships, but excludes passenger ships and service vessels. In total this study includes almost 50,000 vessels. 2. The volume of newbuildings and scrapped vessels are based on forecasts developed by the Institute of Shipping Analysis (SAI). 3. Fuel price trends are based on forecasts by the EIA (US Energy Information Administration) and the IEA (Inter national Energy Agency) and analysis undertaken by DNV Research & Innovation. The uncertainty in fuel prices was analysed based on data from historical prices, available from the EIA (crude oil and LNG/NG) and Clarkson (HFO/ MGO). 4. The technology costs and other assumptions are based on a wide range of sources, ranging from DNV experience data to manufacturers and literature research. 5. The regulations are based on present and future IMO, regional and national requirements. 6. The time in ECA is based on AIS data from Northern Europe and extrapolated to include the North American ECA. The ECA estimates have also been verified against other similar studies. 7. The ship owner’s share of fuel costs and economic preferences are based on a survey conducted by DNV and verified by experience data from relevant projects in DNV. In addition to the above assumptions, we have made additional simplifications. The most important are noted here: 1. Analysis of the world fleet: We chose to exclude some vessel types from the study, and focused on the ‘work horses’ of shipping markets, where technology uptake is most relevant and/or likely, and where the sheer number of vessels is the highest: bulk, tank, container, and offshore supply. Other, ‘smaller’ market segments have not been included, such as passenger ships, ferries, fishing vessels, and many other specialized vessel types. 2. Analysis of modal shifts: The study does not take into account the dynamic shift between different transportation modes – shore/sea in particular. In a more longterm perspective, this would have been a relevant parameter. For example, as short sea shipping is becoming increasingly cost efficient, but with the slow pace of development within this “power shift” between shore and sea, we expect only a minor shift as we move towards 2020. 3. Analysis of cargo handling improvements: Similarly, the study has ignored the fact that some shipping markets are making more progress on the efficiency, and type, of cargo handling – which is making the same vessels more competitive compared to other shipping markets. However, in the context of this study, the impact from these variations is negligible. 4. Analysis of yard and maker capacity: Obviously, the future yard and maker situation will also influence the pace of technology uptake. Yet, somewhat independent of the market development, short or long order books, the “new technology” factor is assumed to be the same. Additionally, it is ship owners who are ultimately deciding in which technology to invest. 5. Availability of seafarers’ competence: The scarcity of competent seafarers will also affect future technology uptake. The fact that we have chosen not to quantify this parameter is probably the simplification which merits the label “wild card”. One case example concerns LNG as a fuel: it takes more than two years to educate a workforce of many thousands, used to working with conventional engines, to switch to “LNG as fuel” engineers. Regardless, we chose to assume that lack of competence will not limit the speed of 50: shipping 2020 technology uptake. We estimate that the industry is able to adapt, and that the next decade will include changes in crews’ competence level. Assumption in scenarios The following assumptions have been made for Scenarios A–D. The assumptions are critical input to the simulations: Scenario Economic Growth: Western world China/India Regulations: Ballast water Sulphur Carbon price Fuel prices: HFO MGO LNG Other characteristics: Size of world fleet (compared to 2012) Design Access to capital A Full steam ahead 4% 8% No BW convention. US regulation in place Global sulphur limit of 0.5% postponed till 2025 No regulation High ($1,150/tonne) 130% of HFO price 110% of HFO price 50–60% larger Standard design High B Knowing the ropes 4% 8% Regional BW regulation covers 80% of merchant shipping. US regulation in place ECA in all coastal areas worldwide. Re-enforced ECA in EU $50-100/tonne High ($1,150/tonne) 150% of HFO price 30% of HFO price (decoupled) 50–60% larger Quick compliance, high level of retrofit High C Sink or swim D In the doldrums 2% 2% 5% 5% BW convention implemented. BW convention implemented US regulation in place but takes time (towards 2020). US regulation in place ECA in all coastal areas worldwide. Re-enforced ECA in EU $30-50/tonne Global sulphur limit of 0.5% postponed till 2020 No regulation Moderate ($750/tonne) 200% of HFO price 110% of HFO price High ($1150/tonne) 150% of HFO price 70% of HFO price (decoupled) 25–30% larger 25–30% larger Limited, high level of retrofit Standard design Low/limited Low/limited Figure 37: Detailed data for four future scenarios. Source: DNV. shipping 2020: 51 52: shipping 2020 Chapter 5: Technology uptake in shipping 2012–2020 More than 50,000 ships have been assessed in the simulation, providing different uptake paths for technologies towards 2020. The results from the model are analysed and discussed in this chapter. They can only give a picture of expected ship owner response given the assumptions provided. Changes to the assumptions will result in different uptakes and the sensitivity of the input is an important part of the result and analysis. This discussion uses the results from the modelling and endeavours to highlight the dynamics and possible consequences of the different assumptions. © Getty Images INDEX •••• Technology uptake in shipping 2012–2020 54 shipping 2020: 53 •••• Technology uptake in shipping 2012–2020 The simulation of the scenarios gives different uptake paths for technologies towards 2020. We ask, which technologies are most likely to be adopted, and when are the turning points? We have analysed almost 50,000 ships towards 2020 and reviewed the technology uptake. The main differences between the scenarios are seen with respect to SOx reduction technologies, where fuel price and regulations play a major role. Scrubbers will be an important technology beginning in 2020, while LNG will have a steady uptake as we move towards 2020, depending on price. With respect to CO2 and energy efficiency, the EEDI will be a major driver and we will start to see ships that are up to 30% more efficient than today’s average vessels. The fleet The fleet assessed in this analysis consists of nearly 50,000 ships for 2012, growing to between 53,000 and 57,000 by 2020, dependent on selected growth scenarios. The fleet is divided into 13 segments, covering cargo carrying ships and offshore service vessels above 100 gt in international trade. How can a ship owner and the industry use the results? The results from the simulation give a picture of expected ship owner response given the assumptions provided. Changes to the assumptions will result in different uptakes and the sensitivity of the input is an important part of the result and analysis. In addition there are several dynamics that are not modelled. First, there are large uncertainties as to what could happen to the MGO price in case of a 0.5% sulphur limit requirement by 2020, which could increase technology demand tenfold. Second, production and installation capacity and the price of a technology such as scrubbers will be highly affected should 10,000 ships decide to install it by 2020. Third, the level of ship gain as a premium charter rate or increase in its probability of charter may vary markedly with low fuel consumption. Fourth, it is uncertain whether there will be a two-tier charter market, and which technologies would give such a benefit. The model determines how each ship owner individually will seek to comply with regulations and increase energy efficiency, and how the resulting macro picture would look. It does not try to optimize the best path ahead, and the individual ship owner reading this report should not necessarily follow this example. The strategy of a ship owner on how to cope with environmental requirements and high fuel prices in the coming decade is dependent on which assumptions the owner thinks are most likely to be realized, how other ship owners are likely to act, and the willingness to take risks. The variety of choices gives the ship owner an opportunity to differentiate. Ropax and passenger vessels are excluded as are fishing vessels, tugs, and other niche segments. Even though these vessels may be prone to a high level of technology uptake in the future, with respect to using LNG as fuel in particular, they have been excluded from the study as this report is focusing on cargo carrying vessels predominantly in international trade. Passenger vessels, in particular cruise vessels, are often at the forefront in embracing new technologies, but they also tend to be a very specialized and heterogeneous group of ships, making it difficult to define a set of “standard” technologies they would apply. Figure 38 illustrates the distribution of vessels in the categories covered by the simulation, and the number of vessels estimated to be outside of simulation coverage. Results The main results from the simulation model are analysed and discussed in the following. Segment Size/sub-type Number of vessels (2011) Tankers Small Medium Large 9,300 100 GT to 60,000 dwt 60,000 to 120,000 dwt over 120,000 dwt Bulk Small 10,000 to 80,000 dwt Large Over 80,000 dwt 8,500 Container Small 100 to 5,000 TEU Large Over 5,000 TEU 4,500 General Cargo 100 GT to 10,000 dwt 16,000 Roro Small 100 GT to 10,000 GT Large Over 10,000 GT 2,100 Offshore Anchor handlers Platform Supply Vessels Others 8,000 Total 48,400 Not included Ropax and passenger vessels Miscellaneous vessels (Fishing, etc) 10,200 Figure 38: Segmentation used in modelling. Source: DNV, 2012. Ballast water The Ballast Water Management Convention (BWMC) has not yet entered into force, but the schedule for mandatory treatment of ballast water is fixed independent of when the convention is ratified. Any delay in ratification will simply create a larger backlog of technology orders. In addition, the US has decided to implement a similar scheme for all ships entering into US waters, which would force a significant part of the world fleet to implement a treatment system irrespective of BWMC progress. The segments covered in this study have mostly medium or large ballast water capacity and, depending on year of build, these ships will have to install a treatment system as soon 54: shipping 2020 The strategy of a ship owner on how to cope with environmental requirements and high fuel prices is dependent on which assumptions the owner thinks are most likely, how other ship owners are likely to act, and the willingness to take risks. as 2012, 2016 or 2018. The requirement to have a system installed is spread out over 2.5 year windows depending on when the intermediate or renewal survey is scheduled, although in reality, the ballast water technology will be installed during an annual dry-dock before this survey. This results in an implementation rate as shown in Figure 39 for Scenarios C and D as only Scenarios C and D assume the BW convention to be implemented. Because the convention is not yet ratified, newbuildings in 2012 and beyond are not currently required to install a system at delivery but, if not installed, they will be part of the fleet that has to retrofit shortly after ratification. The main peak for technology uptake comes in 2017 when the last ships with medium ballast water capacity have to retrofit at the same time as the rest of the fleet has to start retrofitting. After 2019, retrofitting is expected to be largely completed. For Scenarios A and B, where the ballast water convention does not enter into force, there will still be a significant installation of treatment systems, as the US mandates this in its waters, and in B we will see similar regional schemes around the world. The scenarios can be summarised in the following way: A No BW treatment C B D BW treatment for all ships SOx In the coming years, there are two main dates which are important for regulations of SOx emissions: 2015, when the 0.1% sulphur limit is to be enforced in ECAs, and 2020 or possibly 2025, when a global limit of 0.5% sulphur content in fuel will become effective. In addition, regional requirements such as within the EU, may be promulgated. Approximately 40% of the world fleet enters into the North America or Northern Europe ECA during a year, although half of these vessels spend less than 5% of their time there. These are large deep-sea ships primarily transiting the ECA areas to get in and out of port. Only 7% of the ships spend more than 30% of the year in an ECA, many of these smaller tankers and general cargo carriers on short sea trades. When adding to this the fact that only one-third of ship owners pay more than 25% of their fuel bill and that 70% have an investment payback requirement of more than two years, there are very few ships for which the condition for capital intensive solutions such as scrubbers and LNG, is present, at least until 2020, when the global sulphur requirements will make these solutions more feasible. Before 2020, as can be seen in Figure 40, only a small portion of the fleet is likely to invest large sums to reduce SOx. There are two main options for complying with the requirements: (1) using alternative fuels such as distillates or LNG, or (2) using exhaust gas cleaning (scrubbers). The model does not assume any limitation on the availability of either distillates or LNG that could limit the uses of those fuels, nor on the production capacity of scrubbers. In Scenario D, we assume an LNG price that is 30% lower than that of HFO. In this scenario, we foresee that 1,000 newbuildings will be delivered with gas fuelled engines over the next nine years; this equals 10–15% of the expected newbuildings. In the period 2018-2020, about 35% of newbuildings will be delivered with gas fuelled engines. These vessels will have either a pure gas fuelled engine or a dual-fuel engine with the flexibility to run on liquid fuel as well. In addition, approximately 600–700 ships could be retrofitted with dual fuel engines. For Scenarios A and C, with the highest LNG price compared to HFO (110%), the number of installations Number of installations Share of fleet 18,000 16,000 14,000 12,000 10,000 8,000 6,000 4,000 2,000 0 2012 2013 2014 2015 2016 2017 2018 2019 2020 SHIP OWNER PROFILE 100% 90% 80% 70% 60% 50% 40% 30% Retrofit (<1,500 m3; >5,000 m230)% Retrofit (1,500-5,000 m3) 10% Newbuildings 0% 2015-2019 2020 All vessels …spending more than 30% of time in LS area ... and having more than 2 year payback horizon …and paying more than 25% of fuel Figure 39: Projected ballast water treatment technology installations for Scenarios C and D. Source: DNV, 2012. Figure 40: Ship owner investment preferences before 2020, only a small part of the fleet is likely to invest large sums to reduce SOx. Source: DNV, 2012. shipping 2020: 55 are approximately 600–700 where less than 100 are retrofits. In Scenario B, with 70% lower LNG price, the number of gas fuelled ships is over 5,000, with over 1,000 retrofits during the period 2012–2020. Larger vessels will benefit more from running on gas than smaller vessels due to economies of scale in installation and the sheer amount of fuel used by these ships. There are other drivers than the price of LNG for implementing gas fuelled engines, however. In 2020, LNG will be driven by both the 20% EEDI reduction requirement and the global sulphur requirement. The threshold for LNG being a cost-effective compliance option is when a vessel spends about one-third of its sailing time in an ECA. An even lower LNG price can reduce this to 20% of sailing time. CAD B No LNG fuelled ships 5,000 LNG fuelled ships When the global sulphur limit is enforced in 2020, this picture changes as ships would be required to run on low sulphur fuel or clean the exhaust continuously. This will have a significant impact on the implementation of gas fuelled engines, provided the capacity and fuel supply are there, and on scrubbers. Scrubbers may then potentially be fitted to thousands of ships if there is availability and capacity to deliver. In Scenario C, with high LNG price and MGO price, the number of scrubbers in 2020 is close to 20,000, while in Scenarios B and D, the number is 13–14,000. In Scenario A, the global sulphur limit will not come before 2025 and the number of scrubbers is very limited. A BD C No scrubbers 20,000 scrubbers The uncertainty of whether the limit will be enforced in 2020 or in 2025 may delay the use of scrubbers, as ship owners would not take on the additional cost without this being resolved. The EU has signalled that it would enforce a 0.5% sulphur limit in its waters beginning in 2020 irregardless of the IMO review and decision. This will have an impact but not as extensive as a global limit, as most fuel is burned in international waters. Even though there may be a significant uptake of scrubbers from 2020, in Scenario C with the highest LNG and MGO price, which is favourable for scrubbers, there would be only a modest 50 installations annually up to 2019. The main reasons for the limited uptake are (1) that there are few ship owners ready for high capital costs who also spend significant time in ECAs, and (2) that a low LNG price compared to HFO favours investing in gas fuelled engines rather than in scrubbers as this also gives a reduced fuel bill. This is shown in Figure 41. As scrubbers are a new and relatively immature technology for marine use, with limited operational experience outside pilot installations, there are significant uncertainties, particularly on costs and expected cost reduc- tions due to learning effects, which are high in the initial stages of technology innovation. We may see that ship owners will make room for a scrubber in ships built between now and 2019 but defer the installation itself until the technology has matured further and the global sulphur limit is confirmed. However, if this becomes the preferred industry strategy, there is a real risk that technology will not mature sufficiently and that the delivery capacity thus will remain limited with a corresponding increase in system prices at the point of adoption. Most likely, we will see a steady increase of retrofits from 2018 onwards rather than a sharp increase in 2020. The uptake of SOx scrubbers and LNG fuelled engines are, both, very sensitive to developments in fuel prices, carbon prices and technology cost. It would seem that these technology uptake options are competing within the same limited market of ship owners willing to invest. The more favourable the price of LNG is to HFO, the fewer vessels will be delivered with scrubbers. The relative difference between MGO and HFO seems to be of less importance to the uptake of scrubbers, as long as MGO is more than 50% more expensive than HFO. A key element here will be the availability of both LNG and MGO which, at the moment, is uncertain. While these fuels are likely to be available, the cost for marine use remains uncertain. This might also impact the price of HFO which, with less demand, may become cheaper. Fuel price uncertainty and long term expectations will play an important role for ship owners in selecting the right strategy. Number of installations Share of NBs after 2012 70,000 60,000 50,000 40,000 30,000 20,000 10,000 0 Scenario A Scenario B Scenario C Scenario D Pure/dual gas engine SOx scrubber Use of MGO fuel Figure 41: Distribution of SOx reduction options in 2020. Source: DNV, 2012. GAS FUELLED ENGINES CUMULATIVE TECHNOLOGY UPTAKE 2012-2020 FOR NB 18% 16% 14% 12% 10% 8% 6% 4% 2% 0% 2012 2013 2014 2015 2016 2017 2018 2019 2020 10-90% probability Mean Figure 42: Gas fuelled engines: Cumulative technology uptake 2012–2020 for newbuildings in Scenario D. The light blue band denotes the 10–90% probability range. Source: DNV, 2012. 56: shipping 2020 NOx At the moment, there are three main compliance options for the 80% NOx reduction required in Tier III engines: Exhaust Gas Recirculation (EGR), Selective Catalytic Reduction (SCR) and LNG. The choice between the two former is not only a question of cost but also one of system availability and technological maturity. They are currently under development and either or both technologies can reach the tipping point of maturity and become feasible choices for engine manufacturers and ship owners. Most likely, each engine manufacturer will opt for one specific solution, bundled with the engine as a total package. The cost of SCR is slightly higher than EGR at the moment, but technological uncertainty is greater than the cost difference. A clear picture of which of the technologies will be able to succeed in the marketplace will not emerge until ships are being ordered that have to comply with Tier III. In further discussion, we do not distinguish between EGR and SCR, but rather focus on the total number of implementations. DC No ships with EGR or SCR BA 2,500 ships with EGR or SCR LNG is the third option, also having the advantage of fulfilling the SOx requirements and contributing towards improving the EEDI. In the model, when taking an investment decision, the NPV is compared to a set of options complying with both SOx and NOx requirements – e.g. an EGR and a scrubber. In scenarios A and C, approximately 40% of ships built between 2016 and 2020 will have implemented an EGR or SCR system, while in scenarios B and D, the numbers are approximately 30% and 35% respectively. The difference in fuel prices seems to account for this difference. When the price of LNG is low compared to MGO, NOx requirements are met with LNG fuelled engines. Even so, the market share of EGR and SCR is high, with 75% of the shipping needing Tier III compliant engines, which is 40% of the total newbuilding market between 2016 and 2020. Tier III requirements do not seem to be a significant driver for LNG, in and of them. Because of a surge of LNG in 2020, mostly driven by EEDI and sulphur requirements, between 55% and 70% of the newbuildings in 2016–2020 would have Tier III compliant engines. Without a Tier III compliant engine, a ship built after 2016 will not be able to enter the two existing ECAs. The question that remains to be answered is whether a ship owner will opt for a Tier III engine even if the owner initially does not plan to sail in ECA, as the second hand value of the ship might be lower due to the loss of flexibility. CO2 and energy efficiency The mandatory EEDI for tank, bulk, container and general cargo ships which takes effect in 2013, with roll-in roll-out requirements taking effect in 2015, will drive the uptake of energy efficiency measures. In particular, this will be the case beginning in 2020 when Phase 2 of the EEDI kicks in, requiring new ships to be 20% below the IMO reference lines. Options such as LNG and wind power are not strictly energy efficiency measures, but provide less carbon intensive energy to the ships. The results in this chapter refer to CO2 reduction and not energy efficiency. Operational measures, such as trim/draft optimizations, propeller and hull cleaning, weather routing and so on, were not included in this study, which looks only at technical measures. Potential fuel efficiency can be higher given design measures that demonstrate this. In Scenario D, energy efficient designs will gradually improve throughout this decade and a newbuilding contracted in 2020 will, depending on type, emit 10–35% less CO2 than a current but modern ship. The largest reduction in CO2 emissions will be on newbuildings in the tank, bulk and container segments where the EEDI will be an important driver. Offshore ships are not included in the EEDI regulations and fewer measures will be implemented, illustrating that, without the EEDI, less reduction in CO2 will be achieved. However, the modelling does not cover requirements from customers which can be demanding in the offshore sector and drive similar reductions there. Potential cost savings will drive some of the improvements, even with low investment horizons and high fuel burdens. However, the results show that, for 2020 in Scenarios A and C, only one-third of this reduction will Share of new builds CO2 emission reduction 80% 70% 60% 50% 40% 30% 20% 10% 0% Scenario A Scenario B Scenario C Scenario D Pure/dual gas engine EGR or SCR Figure 43: Distribution of NOx reduction options 2016-2020. Source: DNV, 2012. AVERAGE CO2 EMISSION REDUCTION FOR NEW BUILDS 2012-2020 0% 5% 10% 15% 20% 25% 30% 2012 2013 2014 2015 2016 2017 2018 2019 2020 Scenario A Scenario B Scenario C Scenario D Year of build Figure 44: Average CO2 emission reduction for newbuildings 2012-2020. Source: DNV, 2012. shipping 2020: 57 be motivated by cost-efficiency and would be implemented regardless of the EEDI requirements. In Scenarios B and D, this share is higher; approximately half of the reduction is driven by cost. Scenarios C and D are expected to result in the lowest levels of CO2 emissions by 2020 due to low growth, while in Scenario A, the high growth and high price of LNG results in smaller CO2 reductions. The relative uptake of reduction measures on newbuildings does not vary much over the scenarios; even in Scenario C, with low fuel prices, the uptake is more or less the same on newbuildings. At the same time, fewer measures are retrofitted on existing ships in the low fuel price scenario. This enforces the view that the EEDI is an important driver. Scenario B and to some degree Scenario D will have more ships with more than 20% reduction due to the low LNG price and installation of LNG engines. On average, energy efficiency of newbuildings will improve gradually from a 5% reduction in CO2 in 2012 to 10–15% by 2015, and further to 20–25% in 2020. A small number of newbuildings will be very efficient, with a 35% reduction in CO2 compared to 2011, although it will take time before they become a significant part of the total fleet. DC A 3,000 ships >10% CO2 reduction B 8,000 ships >10% CO2 reduction Existing ships will compete against ever more efficient ships, and it remains to be seen if this will create a two-tier charter market where energy efficient ships can command a premium rate or even a higher second-hand resale value. Propulsion efficiency devices and auxiliary system efficiency improvements will have a steady implementation rate on both newbuildings and existing ships. There are few available options for existing ships and these will have limited efficiency improvement in design. This does not preclude operational improvements, but these are outside the scope of this study. The majority of large newbuildings will adopt hull optimization in some way. In order to meet the Phase 1 EEDI requirement in 2015, some ships will opt for smaller engines and lower speed. Those who will use gas fuelled engines to achieve the 20% limit are less likely to de-rate their engine, especially in the scenarios where LNG is a cheaper fuel. To reach the 30% limit, which is not mandatory before 2025, de-rated gas fuelled engines with extensive hull optimization are a likely design combination option. Waste heat recovery will have limited uptake as a technology choice, but this is highly dependent on price. Currently, it is an expensive technology seeing application only in niche segments such as large container vessels, but this technology can be expected to decrease in cost over time. In addition, it can become applicable for smaller vessels as well, as part of an integrated machinery optimization. Air cushion and wind power are technologies in the transition from research to commercial solutions and is in a left end of the S-curve with slow uptake. It is hard to predict the success of these as there are considerable technical and operational risks and more pilots will have to be done before they are put in commercial use. If they are proven to be a practical solution, they may have a large uptake in the fleet. On the other hand, if they are not, they could remain a curiosity. When looking at the financials, neither wind power nor air cushion seems to have a large potential for breakthrough at the moment and both need more time and improvements before that will happen. Figure 45 illustrates how many of the reductions achieved are driven by expected cost savings, based on EEDI or based on both EEDI and cost savings. For example, a reduction measure can have a negative net present value (NPV) but be implemented anyway in order to reach the EEDI requirements. Alternatively, the NPV can be negative but still be needed to reach the EEDI. Finally, the cost-effective measures that will drive the EEDI beyond the requirement are also shown as being implemented. In this example, showing Scenario D, about half the measures have a negative NPV. Fuel mix and demand The fuel mix in 2020 is dependent on how many energy efficiency measures are imple- EEDI reduction Share of vessels AVERAGE EEDI REDUCTION ON NEWBUILDS, SCENARIO D 30% 25% 20% 15% 10% 5% 0% 2012 2013 2014 2015 2016 2017 2018 2019 2020 Beyond EEDI, driven by savings Driven by EEDI and savings Driven by EEDI ENERGY EFFICIENCY GAIN ON FLEET IN 2020 SCENARIO D 90% 80% 70% 60% 50% 40% 30% 20% 10% 0% No change 0-10% 10-20% 20-30% >30% All vessels Ships built after 2012 Ships built after 2015 Ships built after 2020 Figure 45: Average EEDI reduction on newbuildings in Scenario D. Source: DNV, 2012. Figure 46: Energy efficiency gain on 2020 fleet, by year of build in Scenario D. Source: DNV, 2012. 58: shipping 2020 Technology Air cushion Shaft generators Waste heat recovery Gas fuelled engine Smaller engine/ de-rating System efficiency improvement Hull shape optimization Propulsion efficiency devices Number of ships Scenario A Scenario B Scenario C Scenario D 0–5% 5–10% achieved reduction level 10–15% 15–20% 20–25% 25–30% 30–35% <35% 8473 823 9408 599 5827 466 6414 402 2608 1632 299 4 2648 1945 1353 168 2391 844 218 8 1893 922 361 6 952 25 1495 45 529 4 708 0 The colours indicate which technologies are implemented to achieve that reduction, where green is very likely and blue is unlikely. There are a wide variety of possibilities and combinations. Example: Depending on scenario, 500 to 1,500 ships have achieved 30–35% reduction in 2020 compared to the average 2011 ship. Of these all (green) have implemented smaller engine/derating, gas fuelled engine and hull shape optimization. In addition about half (grey) have installed system efficiency improvement and about 70% (light-green) a propulsion efficiency device. Which technologies are applied to achieve certain CO2 reduction levels? Source: DNV, 2012. mented, determining the total demand, and which options are chosen to comply with the SOx requirement, determining the fuel type. The assessment does not take into account the supply of these fuels but predicts the uptake as if the fuels are available in the market at the estimated prices. Currently, LNG prices are regional, and are not global as they are for other bunker fuels. The model uses a global price which in some areas will not project as true; there are likely to be regional differences in price. However, the scenarios use different LNG prices ranging from 30% of HFO in Scenario B, to 70% of HFO in Scenario D, to 110% of HFO in Scenarios A and C. These provide a good picture of the fuel mix and technology uptake sensitivity to fuel price. The current global demand for marine distillates is approximately 30 million tonnes annually. In all scenarios, the 0.1% limit in ECAs will increase the demand to 45–50 million tonnes in 2015. However, the largest increase will be in 2020, with the introduction of the global sulphur limit. This marks a huge increase in the need for distillates to 200–250 million tonnes in 2020, except in Scenario A where the sulphur limit is delayed until 2025. Million tonnes Million tonnes LNG CONSUMPTION 2012-2020 35 30 25 20 15 10 5 0 2012 2013 2014 2015 2016 2017 2018 2019 2020 Scenario A Scenario B Scenario C Scenario D FUEL DEMAND AND MIX PER SCENARIO IN 2020 450 400 350 300 250 200 150 100 50 0 Scenario A Scenario B Scenario C Scenario D LNG MGO HFO Figure 47: LNG fuel consumption 2012–2020. Source: DNV, 2012. Figure 48: Fuel demand and mix per scenario in 2020. Source: DNV, 2012. shipping 2020: 59 Share of total fuel use WORLD FLEET FUEL MIX 100% 80% 60% 40% 20% 0% 2012 2013 HFO 2014 2015 LSHFO 2016 2017 MGO 2018 2019 2020 LNG available but the question is: at what price. Extra demand will either have to be supplied by increased refinery capacity or will be absorbed from other sectors. The 200–250 million tonnes of distillates is equivalent to approximately 4–4.5 million barrels per day or 15% of the current world distillate output from refineries. LNG currently lacks necessary distribution infrastructure, and it is unlikely that ships will be built with pure LNG engines without having the supply ensured. However, dual fuel engines will mitigate this risk and will also encourage investment in infrastructure. Over time, this obstacle will be overcome, also considering that the introduction of LNG will be spread over many years giving time for development of the distribution chain. Figure 49: World fleet fuel mix for Scenario D. Source: DNV, 2012. The use of LNG will only have a limited impact on the need for low sulphur fuel, in the short term. We estimate that the demand for LNG will be between 8 and 33 million tonnes in 2020. ability of scrubbers may hinder this in the short term but, over time, a balance will exist between supply and demand of both distillates and scrubbers, in addition to LNG being available as a third option. In 2020, scrubbers will have a large impact on the fuel mix. Ships burning HFO will have to install scrubbers. In Scenario C with LNG prices higher than HFO and very high MGO prices, the HFO demand will be approximately 125 million tonnes, used by ships with scrubbers on board. In Scenarios B and D, the HFO consumption is 83 and 94 million tonnes, respectively. The capacity and avail- A CDB No ships on destillates 40,000 ships on destillates The supply side of the fuel has not been covered in the analysis but will be a significant factor contributing to the uncertainty of these estimates. Distillate fuel will most likely be Sensitivity analysis The cost-effectiveness of a measure is closely linked to the ship owner’s fuel burden and investment horizon as well as to the fuel price. Changing these parameters could make a substantial difference in technology uptake. The learning effect on the cost of measures will also impact capital costs for ship owners and aid in accelerating uptake. The effect of investment horizon, fuel burden and the learning effect were investigated by running two extra simulations using Scenario D as a base scenario. Briefly: Scenario D presumed an LNG price at 70% of HFO; a global sulphur limit of 0.5% in 2020; and low economic growth. The first extra simulation increased both investment horizon and fuel burden, while the second extra simulation Total number of installations in 2020 Share of newbuildings COMPARISON OF LNG UPTAKE AND LNG/HFO PRICE 10,000 9,000 8,000 7,000 6,000 5,000 4,000 Scenario B Scenario D+ 3,000 2,000 1,000 Scenario D A, C 0 30% 40% 50% 60% 70% 80% 90% 100% 110% 120% LNG/HFO relative price Figure 50: Sensitivity of LNG price, investment horizon and fuel burden on uptake of LNG fuelled vessels. Scenario D+ denotes increased investment horizon and fuel burden. Source: DNV, 2012. SHIPS WITH SMALLER OR DE-RATED ENGINES 100% 90% 80% 70% 60% 50% 40% 30% 20% 10% 0% 0 Scenario C 2015– Scenarios A, D, D+ 2015– Sc2e0n1a2r–i2o0D14+ Sce2n0a1r2io–s20A1–4D+ Scenario B 2015– 200 400 600 800 1000 1200 1400 1600 1800 2000 HFO price Figure 51: Ships with smaller or de-rated engines. Sensitivity of fuel price on uptake of smaller or de-rated engines. Scenario D+ denotes increased investment horizon and fuel burden. Source: DNV, 2012. 60: shipping 2020 No ships with EGR or SCR 3,000 ships >10% CO2 reduction No LNG fuelled ships No scrubbers D+ Dnl D D Dnl Dnl D Dnl D fuelled newbuildings in 2012-2020 plotted against the average relative difference between the LNG and HFO price. For each 2,500 ships with EGR or SCR year and run, the price of each is determined according to the stochastic model. In Scenarios B and D, the prices are decoupled giving a D+ 8,000 ships >10% CO2 reduction different price difference in each run, while in Scenarios A and C, the relative difference D+ 5,000 LNG fuelled ships is constant. This gives us the opportunity to investigate more closely the relationship D+ 20,000 scrubbers between price and uptake. No ships on destillates D+ D Dnl 40,000 ships on destillates Figure 52: Comparison of Scenario D when removing the learning effect (Dnl) and with increased fuel burden and investment horizon (D+). The removal of the learning effect reduces the uptake of scrubbers and LNG, while the increased fuel burden and investment horizon increases the use of these technologies. The other differences are small. removed the learning effect. Additionally, the difference between the fuel prices given in each of the four scenarios is used to investigate the impact of this factor. Investment horizon and fuel burden Increased fuel burden is either the result of changing contracts between ship owners and charterers or reflects a changing business model of the trade, with less spot-pricing and more contracts of affreightment. Investment horizon is more connected to the market outlook of the ship owners, but longer charter contracts usually enable longer investment horizons. In Scenarios A–D, the average investment horizon was defined as approximately 3.5 years and the average fuel burden approximately 30% – meaning that the ship owner pays the fuel bill 30% of the time or trips. To do a sensitivity analysis of these two parameters, the investment horizon is increased to 6.3 years and fuel burden to 65% for Scenario D. The following results can be observed: For NOx technologies, the implementation of EGR and SCR is reduced as more LNG engines are installed, and they increase from approximately 1,700 installations to nearly 6,000 by 2020. For SOx, in addition to LNG as fuel, scrubbers are also more popular with increased investment horizon/fuel burden. The number of installations is doubled from approximately 14,000 to over 30,000. The uptake of energy efficiency technologies on newbuildings will be higher, and more than half will be driven by economic reasons rather than by EEDI requirements. However, with the limited amount of retrofit options available, the introduction of a large number of scrubbers with increased fuel consumption capability, and the fact that a small amount of the fleet in 2020 will be built between 2012 and 2020, these changes would not lead to lower fuel consumption in 2020 compared to base Scenario D. However, the shift to LNG will lower the CO2 emission by 2 percent. Learning effect The learning effect has a significant impact on new technologies. Without this, the implementation of scrubbers would decrease from the projected 14,000 to fewer than 6,000 in 2020 in Scenario D. The impact of the learning effect on gas fuelled engines was not as severe, decreasing the number from 1,700 to 1,100. For energy efficiency measures the difference was minimal, most likely because many of these measures are more mature, the learning effect is already achieved, and many implementations are driven by requirements. Fuel prices If we look at the number of LNG fuelled engines compared to the LNG price, we see a clear trend towards an increasing number of installations with lower LNG prices relative to HFO. Figure 50 shows the share of LNG Increasing the investment horizon and fuel burden shifts the implementation curve upwards as expected. The difference is significant. The curve for Scenario D is similar to the uptake for Scenarios A and C, while for Scenario B, the curve seems to shift upwards. There are other parameters that are changed in the scenarios, making it difficult to isolate the impact of factors. The difference between Scenarios A and C is the introduction of a global sulphur limit in 2020, lower fuel prices in general, and a higher discount rate in Scenario C. These will all work for or against LNG as a fuel, and the result is a slightly lower number of installations in Scenario C. For Scenario B, the discount rate is lower than in D. In addition, the learning effect might explain its shift upwards in number of implementations. For energy efficiency measures, the EEDI is a strong driver. A closer look at the uptake of smaller or de-rated engines (Figure 51) shows that there is a slight increase in the uptake of these solutions with approximately 20% of newbuildings when the HFO price is $600/ tonne, and up to 30% with a price of $1100/ tonne. Using an increased investment horizon and fuel burden, the uptake is approximately 50 percent. In 2015, when Phase 1 of the EEDI starts, the uptake shifts up to 70-80 percent. In Scenario C, the fuel price level is lower, but still the uptake is at the same level as in the other scenarios. In Scenario B, the LNG price is very low, many ships are built with LNG fuelled engines, and these ships are less likely to implement a smaller engine, both because the fuel cost is lower and they have already met the EEDI requirements. shipping 2020: 61 62: shipping 2020 Chapter 6: Trends and drivers beyond 2020 In this study, we have focused on the technologies that are likely to be adopted between 2012 and 2020. However, technologies will continue to evolve, mainly as a response to emerging regulations, fuel prices and commercial needs. In this closing chapter, we share with you some of our thoughts about how we see the world changing and what implications this could have for the future of shipping beyond 2020. © Getty Images INDEX •••• Trends and drivers beyond 2020 64 shipping 2020: 63 •••• Trends and drivers beyond 2020 As this report has analysed technology uptake through 2020, we conclude this document with some thoughts on technology adaptation beyond a 2020 perspective. The maritime industry will be faced with ever increasing requirements for safety, security, environmental and efficiency performance beyond 2020. Environmental and efficiency demands will remain front and centre for technology development, with the IMO global sulphur limit and the EEDI being the strongest drivers. This may lead to fundamental changes in the industry. that the technology solution to one issue does not unduly constrain choices addressing the others. A fine balancing act will be required, in particular when generally increasing fuel prices, high investment costs, the potential lack of financing and probably soft charter rates are factored in. As the world population and economy grows and the centres for economic activity shift, the demand for shipping will increase and we will see a corresponding shift in trade patterns to meet the changes in demand. High growth economies will drive demand for bulk and tank shipping, as well as, increasingly, containerized goods. Coastal shipping will increase as a consequence of shore-based megacities, congestion on land, and a general demand for regional transport of goods and people. Developing countries, such as Brazil and Indonesia, will become the driving economic forces in the world as China advances in its development path. At the same time, there will be a need to develop infrastructure in developing countries in order to facilitate direct calls for larger vessels. The development of North-South trade routes may impact container shipping networks, with ports in West Asia or Africa increasing their transhipment quotas. Fuel Fuel will remain expensive beyond 2020 and will drive demand for energy efficient ships. These will focus on optimal energy use, and will be designed and operated with alternative fuels such as LNG, power systems, and light weight construction. The demand for renewable energy will have grown significantly and this in turn will create new markets for the maritime sector, including shipping of biofuels. In order to serve offshore power infrastructure development and operation, new specialized ships will be required. Regulations There are numerous environmental issues emerging on the agenda that are set to become important after 2020. Those expected to be most significant from a regulatory perspective are black carbon, hull bio-fouling and underwater noise. Black carbon emissions from shipping may turn out to be a significant contributor to global warming and/or polar ice melting. The science is still not clear, but it is an area in which extensive research is ongoing, and it has entered the IMO agenda. Hull bio-fouling is recognised as a major transport pathway for alien species of the same order as ballast water. The IMO has developed a voluntary guideline to manage this, and it would not be unexpected if this transformed into regulations over the next 10–15 years, with potentially significant cost implications. Underwater noise is also raising increased attention in recent years due to its possible impact on ocean-dwelling mammals. While the science remains unclear, regulatory initiatives and public sentiment may be building towards international regulation. Applying noise control measures to ship engines, hulls or propellers would be technically challenging and also, likely, expensive. Implications Considering the issues above and their interaction with each other, it should be apparent that navigating the regulatory landscape to decide on appropriate technical solutions is not a trivial task. Addressing SOx, NOx, ballast water and energy efficiency requirements more or less in the same timeframe requires a careful balancing act, where care must be taken so It is also likely that the realities of climate change will motivate the regulatory landscape to develop more comprehensive and stringent regulation of greenhouse gas emissions beyond 2020 and, as a result, there will be a growing demand for “clean” shipping technologies in order to comply cost-effectively. The EEDI will enter its last phase in 2025 with a 30% emission reduction requirement. The development of new designs will determine if this is the lowest level or if a phase 4 will be implemented. Climate change and its potential impact on the offshore industry, as well as on ship design and operations, will remain subjects of much debate. A shift in wave patterns, increased wave heights, and more severe weather conditions will be observed, resulting in a call for enhanced safety standards. A possible shift towards risk-based regulation will facilitate increased innovation in ship design and the exploitation of novel solutions. Regulatory initiatives on a regional, national and local scale could also have a large impact on the maritime industry. Innovation and adoption of new solutions In DNV Technology Outlook 2020, we highlight six key areas that offer a significant potential for innovation and that we believe will be at the core of R&D in the industry in the next decade: 1. The low energy ship: Multifunctional ship types and/or technological advances in drag reduction, propulsion, and materials are expected to herald new ship concepts. With the significant efforts already being put into the making of more energy efficient ships, as has been observed only during the past 1–2 64: shipping 2020 © DNV Quantum – a container ship concept for the future Quantum has been designed to transport more cargo while using less fuel and with reduced environmental impact. The focus has been on flexibility in a future of uncertainty, while also ensuring efficient and reliable operations. Source: DNV. shipping 2020: 65 years, one should expect the years between now and 2020 to bring further progress. 2. The green fuelled ship: Stricter environmental regulations that require reduced emission levels of SOx, NOx and particulate matter are pushing the shipping industry towards further use of cleaner energy sources. Abatement technologies, such as exhaust gas recirculation, scrubbers, and catalytic reduction, can typically meet some of these regulations. LNG and biofuel blends will also be further exploited beyond a 2020 perspective. 3. The electric ship: “The Prius of the Sea” could contain diesel-electric configurations, marine fuel cells, battery packages, solar panels or retractable wind turbines, and compact superconducting motors. These powering systems will require design, operation and control of energy production and conversion in an integrated manner, and more complex systems will be manufactured. 4. The digital ship: While ‘e-Navigation’ is not new to shipping, we can expect that, by 2020, the majority of the fleet will have adopted e-Navigation technologies. Onboard electronic charts will become the unifying platform on the digital ship, integrating and visualising information from other applications in related areas such as security and navigation risk, port entry, weather routing, and similar needs. 5. The Arctic ship: The next decade will see an increase in Arctic ship traffic. This will lead to faster development of Arctic-related technologies, such as ice route optimization software, hull load monitoring systems, and, possibly, the introduction of new ice breaking concepts. The shipping industry is at the forefront when it comes to developing solutions for tomorrow’s vessels, vessels that will fulfil current and coming requirements related to energy efficiency and environmental performance. What we have learned through Technology Outlook 2020 and through the work on this Shipping 2020 report is that getting to a stage with several full scale demonstrator projects is key, as is governmental support of R&D. The best way to convince a traditionally conservative industry is to demonstrate full scale performance, and to present a convincing cost-benefit case based upon similar results. When it comes to diffusion, or technology uptake, it has been an historically slow process and will continue to be so. The most important stakeholder to make decisions with respect to new technology will continue to be the ship owner. The industry will probably continue to produce cost-benefit calculations demonstrating that technology ABC is costbeneficial in a one-, five-, or perhaps ten-year perspective, but ship owners will still be reluctant to invest in that particular technology. Why? Again, underlying factors, such as lack of capital, uncertain market outlook, and technical risk aversion will continue to make it difficult to predict “optimal solutions” for the ship owner. Thus, what the analysis shows is not necessarily what the industry will do. In the coming years, there will be ample opportunities to select a differentiating strategy to move ahead of competitors. 6. The virtual ship: In order to manage the inherent risk in innovative solutions, there will be a further drive towards use of modelbased techniques for assessing novel concepts and technologies with respect to technical and economic performance of ships, from a life cycle perspective. Whilst all of these solutions offer great promise, the shipping industry is still faced with two main challenges: technology development, and diffusion. 66: shipping 2020 References and sources • AIS-data for Baltic and North Sea, 2010. • Bass, Frank M.: http://www.bassbasement.org/BassModel/Default.aspx, 2012. • Buhaug, Ø., Corbett, J. J., Endresen, Ø., Eyring, V., Faber, J., Hanayama, S., Lee, D.S., Lee, D., Lindstad, H., Mjelde, A., Pålsson, C., Wanquing, W., Winebrake, J. J., Yoshida, K.: Updated Study on Greenhouse Gas Emissions from Ships: Phase I Report; International Maritime Organization (IMO) London, September 2008. • DNV, Maritime Fuel Price and Uptake Projections to 2035: DNV Research & Innovation, 2012. • IEA/OECD, 2000: Experience curves for energy technology policy, 2000. • IHS Fairplay, World Fleet Database, 2012. • North European LNG Infrastructure Project, 2011, A feasibility study for an LNG filling station infrastructure and test of recommendations. • OECD website: www.oecd.org, 2012. • Review of Maritime Transport 2010, United Nations Conference on Trade and Development (UNCTAD), 2010. • Stopford, M., Maritime Economics, 3rd ed., Routhledge: London, UK, 2009. • The SAI Shipbuilding Markets Forecast, February 2012. • The SAI Global Shipping Market Report, February 2012. • World Bank, Global Economic Prospects, Uncertainties and Vulnerabilities, January 2012. DNV services If you have further questions about what DNV can help you with, please contact your local CSM or the closest DNV office. The following is a list of the most relevant services on environment: Fuel saving and energy efficiency • Ship Energy Efficiency Management Plan advisory • Energy Efficient operations • Hull optimization and concept evaluation • Machinery optimization and concept evaluation • Performance management • Ship Energy Audit • Fuel Quality Testing • Maritime Transport System Improvement Decision support on future fuels, designs and technology • Evaluating options for the next decade of energy efficient design and operation • Risk evaluation and mitigation of new operational environments • Technology screening • Technology Qualification Regulatory compliance • Statutory Certificates (IEEC, BWMC, IAPP, EIAPP, etc) • Approval of Exhaust Gas Cleaning Systems • Type Approval of Ballast Water Treatment Systems • Inventory of Hazardous Materials and Asbestos Statement • Recycling Yard Services • Development of Management Plans (BW, SEEMP, VOC, etc) • Preparation of Energy Efficiency Design Index Technical file Standards and ratings verification • Triple-E rating • Clean Shipping Index Verification • ISO Certifications (i.e. 14001, 50001) shipping 2020: 67 Det Norske Veritas AS NO-1322 Høvik, Norway I Tel: +47 67 57 99 00 www.dnv.com THIS IS DNV DNV is a global provider of services for managing risk, helping customers to safely and responsibly improve their business performance. Our core competence is to identify, assess and advise on risk management. DNV is an independent foundation with presence in more than 100 countries. © Det Norske Veritas AS 08-2012 Design: CoorMedia.com 1201-073 Printing: 07 Oslo as 3,000/08-2012 Cover photo: Getty Images
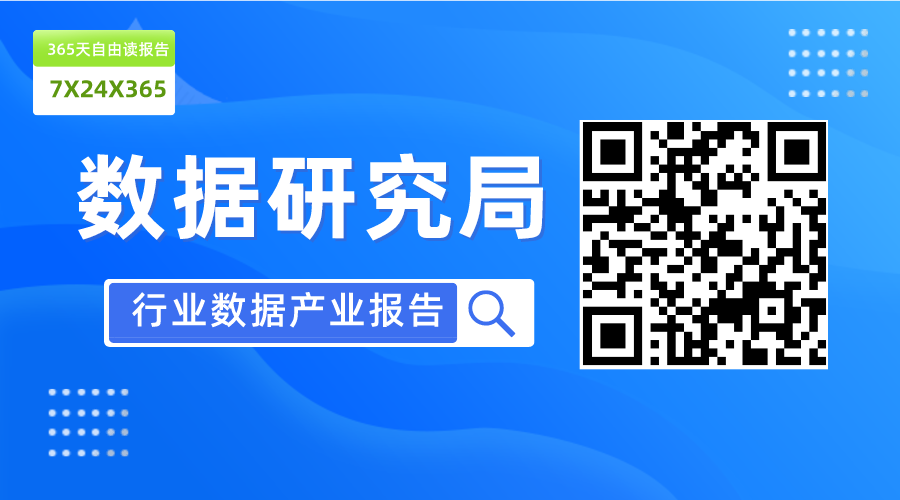
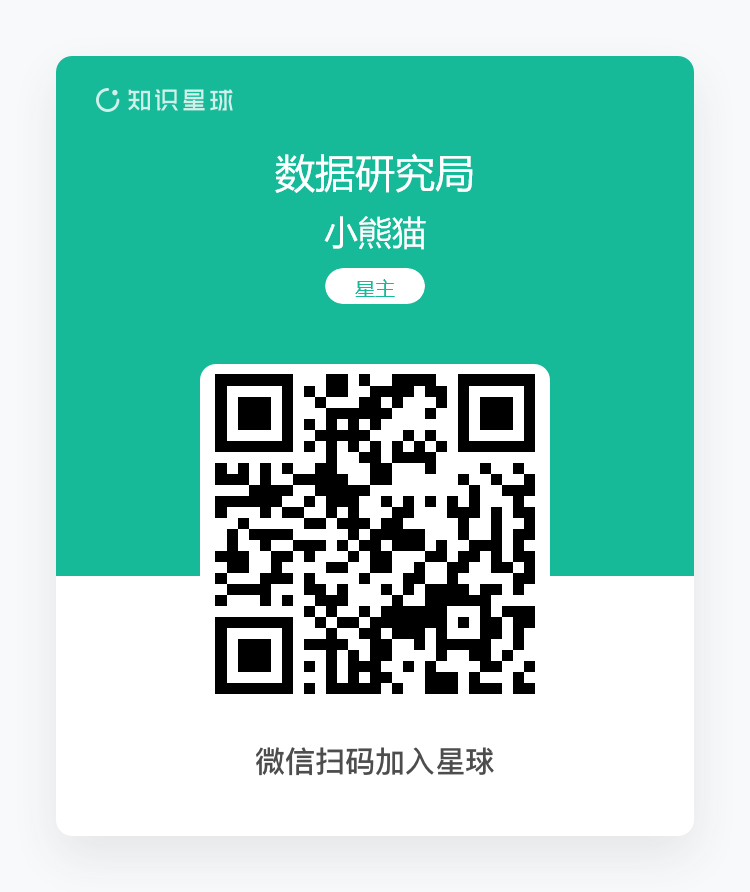